the Creative Commons Attribution 4.0 License.
the Creative Commons Attribution 4.0 License.
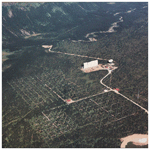
History of the Tromsø ionosphere heating facility
Michael T. Rietveld
Peter Stubbe
We present the historical background of the construction of a major ionospheric heating facility, “Heating”, near Tromsø, Norway, in the 1970s by the Max Planck Institute for Aeronomy; we also detail the facility's subsequent operational history to the present. Heating was built next to the European Incoherent Scatter Scientific Association (EISCAT) incoherent scatter (IS) radar facility and in a region with a multitude of diagnostic instruments used to study the auroral region. The facility was transferred to EISCAT in January 1993 and continues to provide new discoveries in plasma physics and ionospheric and atmospheric science to this day. It is expected that Heating will continue operating along with the new generation of IS radar, called EISCAT_3D, when it is commissioned in the near future.
- Article
(6379 KB) - Full-text XML
- BibTeX
- EndNote
In the following, we present the history of a major ionospheric research facility that played a very important part in both of the authors' scientific careers. The second author was involved right from the start of the project until the transfer of the facility from the Max Planck Institute for Aeronomy to the European Incoherent Scatter Scientific Association (EISCAT) in 1993. The authors worked together for several years up to this date, after which the first author managed the facility until his retirement in 2020. This history, which concentrates on the administrative and technical aspects but also mentions important scientific collaborations and results, is based on the authors' personal memories and documents that are available to them but which may not be easily accessible to all readers.
The history of ionospheric heating experiments started in the early days of radio with the Luxembourg effect (Tellegen, 1933): the modulation of a powerful radio transmitter was imparted in the ionosphere on another radio wave transmission. The explanation was that the powerful radio wave could heat the free electrons in the plasma which makes up the ionosphere and, thus, change the properties of the medium. The ionosphere is the ionized part of the upper atmosphere, extending from about 70 km to several hundred kilometres. By injecting high-power radio waves into this plasma, it became possible to use the ionosphere as a plasma laboratory without the restriction of boundary walls which limit some laboratory plasma experiments. Through these experiments, a better understanding of plasma physics was made possible and a new technique to learn more about the ionosphere itself was provided. A major advance was made in the early 1970 s with a heating facility in Boulder, Colorado, USA, showing a plethora of unexpected ionospheric phenomena that could be triggered by powerful high-frequency (HF) waves (as outlined in Utlaut, 1974). This spawned international interest, especially in the Cold War era, during which time radio communication via the ionosphere was still important and ionospheric irregularities played an increasingly important role in understanding radar echoes from natural phenomena as well as synthetic objects. At the same time, the incoherent scatter (IS) radar technique for measurement of ionospheric plasma properties was developing rapidly, with US facilities in Arecibo (Puerto Rico) and in Millstone Hill and Chatanika (Alaska), and European radars in France and the UK either operating or being planned. An advanced tristatic European radar was also in the planning for northern Scandinavia to investigate the auroral ionosphere. The Max Planck Society, one of the major scientific research organizations in Germany, was already a partner in the planning of this radar and became one of the six associates in the European Incoherent Scatter Scientific Association (EISCAT). Two Max Planck institutes were involved: the Max Planck Institute for Extraterrestrial Physics, in Garching, and the Max Planck Institute for Aeronomy (MPAe), in Katlenburg-Lindau (now the Max Planck Institute for Solar System Research, in Göttingen) (Haerendel, 2016). Ian Axford, who had just been appointed as the new director of MPAe in 1974, strongly supported and influenced the development of EISCAT and suggested building an ionospheric heating facility. About this time, similar facilities were being built near Arecibo and in the former Soviet Union, both at low to mid-latitudes. The MPAe had a long history in the area of HF radio research and techniques for the purpose of studying the ionosphere and upper atmosphere (e.g. Czechowsky and Rüster, 2007); therefore, designing and constructing a high-power HF transmitting facility was well within the competence of the institute. With the new director (Axford) came a number of international guest researchers who helped develop the science case for an ionospheric heater as well as advising on technical issues. Some of these were Fred Hibberd from Australia, Jules Fejer from the USA (who was also an external scientific member of MPAe), and Dick Dowden from New Zealand. The project was led by Prof. Peter Stubbe and Dipl. Phys. Helmut Kopka (Dipl. Phys. stands for the German degree of Diplom-Physiker, which is approximately equivalent to a masters degree in physics). Other scientists from MPAe who were closely involved with “Project Heating”, as it was called, were Prof. Harry Kohl, Dr. Gerhard Rose, and Dipl. Phys. Hans Lauche.
The planning and design of Project Heating started in 1975. The total cost of the facility was estimated to be 6 million German marks (DM) at that time, equivalent to EUR 3 million. Approximately one-third of this amount (for development, operating, and personnel costs) came from the operating budget of MPAe, one-third was from an investment from the Max Planck Society, and the remaining one-third was from an investment from the German Research Foundation (Deutsche Forschungsgemeinschaft – DFG). The amount did not include the salary costs of the staff who worked on the project at MPAe. The project was formally a joint project between MPAe and the University of Tromsø (UiT): funding, design, and construction were the responsibility of MPAe, while UiT, especially Asgeir Brekke and Reidulv Larsen, arranged local permits and infrastructure, such as the buildings. UiT was also the main Norwegian partner in EISCAT (see Holt, 2012). For several reasons, the Heating site was chosen to be next to the new EISCAT radars in Ramfjordmoen, about 30 km south of Tromsø by road. MPAe and UiT were already involved in the EISCAT radar project, which would be a major diagnostic for Heating, and there were many common infrastructure items, such as communication and the electric power line that was specially installed to supply both projects. An important feature of the location in the Arctic as compared to mid-latitudes or low latitudes is that the Earth's magnetic field is close to vertical; this is advantageous for studying many plasma physical phenomena. Figure 1 shows an aerial view of the Heating facility next to the EISCAT radars. A history of the EISCAT system was recently published by Wannberg (2022).
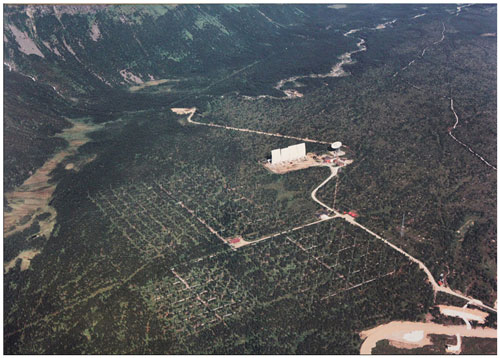
Figure 1Aerial photo of the Heating antennas and IS radars at the Ramfjordmoen site from 1996. (Photo credit: Fjellanger Widerøe AS)
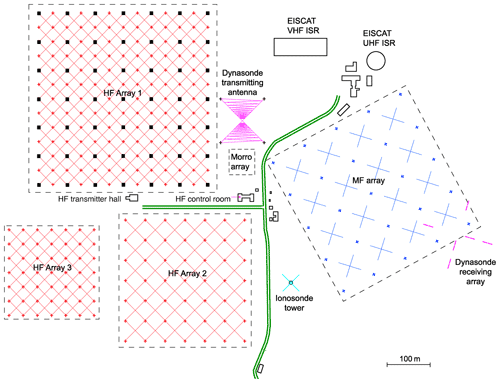
Figure 2Schematic diagram showing the layout of antennas and major infrastructure at the Ramfjordmoen site (to scale). The red diagonal lines in the HF arrays represent the full-wave antennas attached to wooden masts at each end (small red crosses). The feed points of each crossed-dipole antenna is where the red lines cross each other. The black squares in HF Array 1 show the original 23 m tall wooden masts to which the low-frequency antennas were attached (until 1985), and the red crosses in Array 1 show the 12 m tall wooden masts added around 1989 for the modified array containing higher-frequency antennas similar to Array 3. The Dynasonde (HF sounder), housed in the same building as the HF control room, and its associated antennas are an integral part of the Heating facility. The EISCAT IS radar antennas and buildings are shown at the upper right. The large blue crosses show the crossed half-wave dipoles of the 2.78 MHz MF (medium-frequency) radar transmitting antenna, suspended between masts (small blue crosses). The ionosonde tower supports the transmitting antenna for the University of Tromsø's digisonde. The Morro array is an antenna for a 56 MHz MST (mesosphere–stratosphere–troposphere) radar from the University of Tromsø. The green double line shows the road, and the unlabelled boxes are buildings or huts with optical and other instruments.
Design concepts for the facility were outlined by Kopka et al. (1976), and these were largely adhered to in the final facility. The design of the facility was challenging. To cover the wide frequency range from 2.7 to 8 MHz, three antenna arrays, each of 6×6 crossed full-wave dipoles were built to the same design, but the lengths, spacing and height above ground were scaled by between arrays. The full-wave antennas were rhombically broadened to provide a wider bandwidth than single-wire antennas. The centre frequency was 3.31 MHz for Array 1, 4.71 MHz for Array 2, and 6.63 MHz for Array 3, each with a 37 % bandwidth. Each array was fed by high-power coaxial cables, with a total length of ca. 50 km, from a central building housing 12 transmitters of up to 100 MW each. The gain of each array was 24 dBi at the mid-frequency (dBi is the maximum gain of the radiation pattern in decibel compared with that of an isotropically radiating antenna), resulting in a maximum effective radiated power (ERP) of 300 MW. Figure 2 shows a schematic diagram of the antenna arrays as well as other relevant instruments and buildings in the Ramfjordmoen site. Commercial high-power coaxial cables and other components were too expensive; thus, in-house designed and built air-filled coaxial cables, baluns, power splitters, quarter-wave transformers, stubs, and motor-driven coaxial switches were produced from aluminium pipes and specially designed castings and fabrications (see Fig. 3). This was a highly innovative but also risky undertaking. The design worked very well electrically, but it required retrofitting of an air dryer and compressor to feed dry air into the whole system in order to prevent the ingress of moisture as well as the installation of many more wooden supports under the coaxial lines in order to survive the harsh winter conditions of northern Norway. The accumulation of up to 2 m of snow and ice during a winter could bend, deform, or break some of the aluminium components, and the subsequent thaw in the spring meant that parts of the lines could be under water. Nearly all of the connectors are aluminium, so the prevention of moisture ingress in the transmission lines is very important, which the compressor and dryer achieved successfully. In spite of the air dryer and extra wooden supports under the cables, maintenance of this coaxial cable feed system would remain a fairly labour-intensive annual task each summer.
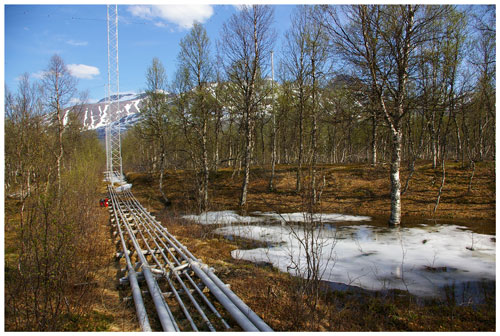
Figure 3The fabricated coaxial cables and towers in one row of antennas of Array 2 in spring of 2010. The vertical tubes, which are part of the tower supporting the antenna, are also coaxial cables acting as quarter-wave transformers supplying the radio frequency (RF) current to the antenna. Array 3 has an identical design but is smaller in size. Originally, Array 1 had similar antennas, larger in size, but these were modified after the storm in 1985. (Photo credit: Michael T. Rietveld)
A conducting ground plane was never installed, as it was felt to be unnecessary given the moist ground conditions at Ramfjordmoen, so the calculated ERP assumed a perfectly conducting ground. In hindsight, a ground plane might have increased the actual ERP, as subsequent modelling reported in Senior et al. (2011) suggests that, using measured values of ground conductivity and dielectric constant, the actual ERP is approximately 75 % of that calculated assuming a perfect ground.
The transmitters were state-of-the-art vacuum tube transmitters with a wideband solid-state driver and automatic tuning and impedance-matching system employing variable vacuum capacitors and switchable inductors between frequency bands. The main power amplifier was a linear, water-cooled Siemens tetrode RS2052CJ vacuum tube with variable voltage power supplies using thyristors. A prototype design from Siemens was used to build the transmitters, including power supplies, in-house. The wideband solid-state driver was designed to deliver ca. 1.5 kW to the power amplifier tube and was built in-house. It was found that the impedance matching to the tube was not good; thus, an impedance-matching filter needed to be designed and constructed, which turned out to be the equivalent of an engineering masters thesis (Diplomarbeit in German). Figure 4 shows some of the open transmitter cabinets and three people who were closely involved in the early part of the project.
The radio frequency (RF) waveform was produced using the new (at the time) HP3325 A synthesizers, with one for each of the 12 transmitters and an additional one as phase reference. These were controlled by computer from a Commodore PET (personal electronic transactor) microcomputer running a combination of assembler and BASIC (Beginners' All-purpose Symbolic Instruction Code) language programs with a specially developed interface to the essential transmitter hardware. For modulation of the transmitter output, another microcomputer, a Texas Instruments TM 990 programmed in assembler language, was used with 12 digital-to-analogue converters and other special hardware to provide external control voltages to the HP synthesizers to vary their amplitude and phase. Low-frequency synthesizers could also be used to amplitude modulate the RF waveform from the HP synthesizers. Figure 5 shows a block diagram of the transmission system and the power distribution to the various antenna arrays.
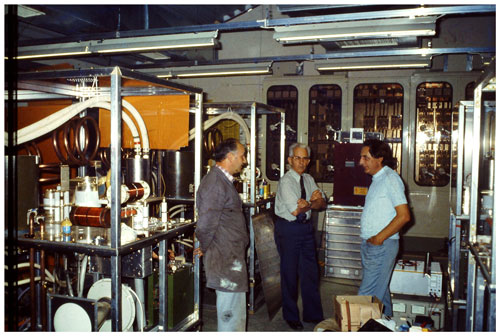
Figure 4Three important people involved in the construction of Heating. The photograph shows, from left to right, technician Helmut Gegner and scientists Jules Fejer and Helmut Kopka in the transmitter hall in 1979. Four open 100 kW transmitters are visible, which were used in the first experiments. (Photo credit: MPAe)
Construction of the facility in Ramfjordmoen occurred between 1978 and 1980. This involved long periods of work by teams of up to about 10 engineers, technicians, and other workers from MPAe, who stayed in the main accommodation and control building in Ramfjordmoen. In anticipation of large teams having to stay for long periods, this building was designed to be relatively spacious and well equipped. The MPAe staff who designed and built parts of the facility were Dr. Rainer Kramm (electronics and software), Richard Zwick, and Lothar Bemmann. Heinz-Günther Kellner, Eberhard Schäfer, Karl Schreiber, Rudi Pabst, and Willi Butscheck built and assembled much of the hardware, and Helmut Gegner and Klaus Eulig assembled, maintained, and operated the facility for the duration of the project under MPAe ownership. Many other workers from MPAe were involved in the construction of parts in Katlenburg-Lindau and the subsequent assembly work in Tromsø for shorter periods.
In the control building, an advanced HF radar developed at the National Oceanic and Atmospheric Administration (NOAA, Boulder CO), called the “Dynasonde”, was also installed. This instrument was and still is essential to determine the state of the ionosphere continuously and in real time, independent of the EISCAT IS radars, which are not always operating. It was also an important diagnostic instrument to measure the effects of heating the ionosphere. J. W. (Bill) Wright and R. (Dick) Grubb were valuable collaborators in the set-up and use of this versatile instrument. The computer hardware and operating and analysis software were later upgraded such that this instrument is still providing advanced high-quality ionospheric data to this day (Rietveld et al., 2008).
There was an official inauguration in September 1980, by the director of MPAe, Ian Axford, and the rector of the University of Tromsø, Prof. Yngvar Løchen; guests from the USA, Bill Gordon and Tor Hagfors (the latter of which was the director of EISCAT at this time), who were both pioneers in the ionospheric heating field, were also present. At this stage, there had already been experiments performed using the available, finished transmitters out of the final 12 transmitters. The first experiments were carried out with the Norwegian partial reflection (PRE) system of UiT (August 1979), followed by the first very low frequency (VLF) excitation experiments with Dowden's system (March 1980), the excitation of micropulsations with Hans-Joachim Lotz and Jürgen Watermann (September 1980), and the first anomalous absorption experiments with Tudor Jones' group from the University of Leicester, UK (October 1980). These first experiments are described in summarized form in Stubbe et al. (1980). Another early result from August 1980 was the measurement of fast attenuation of the reflected HF wave which was explained in terms of the parametric decay instability and a slower attenuation by heater-induced striations (Fejer and Kopka, 1981). The original duration of Project Heating was limited to the end of 1987 but, because of the successful results obtained, it was decided to extend the project until the end of 1992.
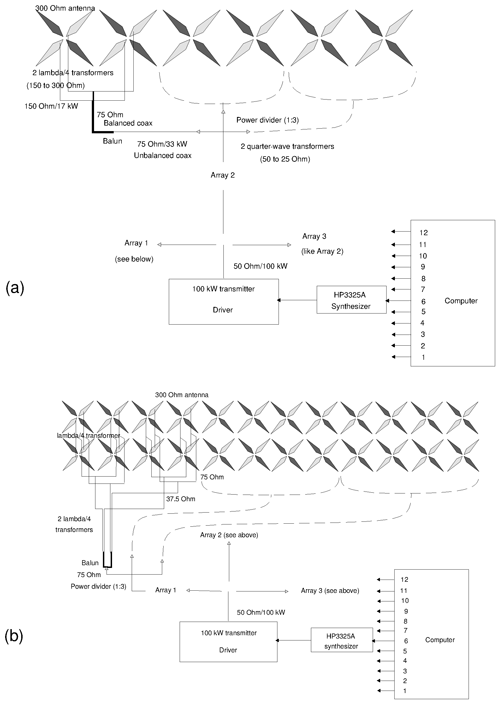
Figure 5Diagram showing the transmission system and how the power was distributed from one transmitter (a) to six crossed dipoles in each of the antenna arrays before the storm in 1985 and (b) to 24 crossed dipoles in the rebuilt Array 1 from 1990. After 2008, the HP3325A synthesizers were replaced by direct digital synthesizer boards.
A comment is in order about the name of the facility. At MPAe, it was a project, simply called “Heating” because of the main physical effect of heating the electrons in the ionosphere that it could produce. This was not an acronym, although it has sometimes been spelled with all capital letters. After the transfer to EISCAT, it was also called Heating or the Heating facility. Because thermal heating of electrons is not the only effect of the powerful HF wave, it is sometimes simply called the HF facility or HF pump because the HF waves can excite and pump various plasma instabilities, thereby energizing electrons to suprathermal energies.
The results of the first 2–3 years were quickly published and summarized in a review paper by Stubbe et al. (1982), followed by another review 3 years later (Stubbe et al., 1985). Many important scientific discoveries were made in the first 10 years of operation. One of the highlights was the exploration of the process by which ionospherically induced currents in the lower ionosphere (ca. 70–110 km) generate extra low frequency (ELF) to very low frequency (VLF) radio waves in the audio-frequency range and below. With measurements from ELF/VLF and micropulsation receiving systems installed by R. L. Dowden from Otago University, New Zealand, at a UiT field station in Lavangsdalen, about 17 km from the heater site, along with theory and modelling, the authors could explain the characteristics and mechanisms involved in the generation process of these low-frequency waves. The first author became involved in these experiments as a post-doctoral scientist in 1981 after completing a doctoral degree on VLF wave research at Otago University. ELF/VLF wave propagation experiments were also performed with satellite receivers in the ionosphere and magnetosphere and (unsuccessful) attempts at conjugate wave reception at the Australian Antarctic base in Mawson. Later collaboration with Richard Barr from New Zealand explored Earth–ionosphere waveguide propagation and the evaluation of different modulation techniques to explore the efficiency of such wave generation, both experimentally and with modelling.
Lavangsdalen was also the site of the accidental discovery of another important phenomenon – stimulated electromagnetic emissions (SEE) – consisting of the generation of secondary HF waves due to plasma processes in the ionosphere under the action of the powerful pump wave (Thidé et al., 1982; Stubbe et al., 1984). This discovery opened up a major research area at all heating facilities (e.g. Leyser, 2001).
Early experiments were also performed with the local partial reflection experiment (PRE) from UiT as well as HF diagnostics from the group at the University of Leicester led by Tudor Jones. Research from this group led to many theses and papers, such as those by Terry Robinson, Alan Stocker, and Farideh Honary. Naturally, the EISCAT UHF (ultra-high-frequency) radar and, later, the VHF radar when it came on line in 1985, were used as a new diagnostic instruments. Much scientific interest was directed at IS radar experiments and Heating, where the Earth's magnetic field was near-vertical, in contrast to the interesting results coming out of the Arecibo facility, where the magnetic field was near 45∘. For near-field-aligned HF pumping, the Langmuir turbulence results were expected to be much stronger and, indeed, the experimental results exceeded expectations and provided a source of controversy. There was fruitful exchange between theory and experiment from these experiments. For these IS radar diagnostics, which usually involved high time and spatial resolution observations of strong coherent signals induced in the ion and plasma line spectrum, special modulations and detection algorithms had to be developed with very different requirements from the usual IS measurements of the undisturbed plasma. These programmes were developed mostly by Harry Kohl and Terrence Ho from MPAe. Collaborations were developed with researchers from many countries. These included Tor Hagfors, Brett Isham, Cesar La Hoz, Frank Djuth, Mike Sulzer, and Shanti Basu. There were several cooperative projects with scientists from the former Soviet Union in various institutes such as the Polar Geophysical Institute in Murmansk, IZMIRAN (Institute of Terrestrial Magnetism, Ionosphere and Radiowave propagation) near Moscow, and the Institute of Radio Astronomy in Ukraine. Some campaigns after 1990 involved scientists bringing diagnostic instruments with them from the former Soviet Union, which was impossible in the days of the Cold War.
From the start of Project Heating, it was planned to fly rocket instrumentation through the heated region (Stubbe et al., 1978) from the Norwegian rocket base at Andøya. The principle investigator for this HERO (HEating ROckets) project was Dr. Gerhard Rose from MPAe, with collaborators from the Fraunhofer Institut für Physikalisch Messtechnik (Institute for Physical Measurement Techniques) Freiburg, Germany; the Norwegian Defense Research Establishment; and the University of Oslo. The HERO project was the first project especially designed to undertake in situ measurements of the HF-generated Langmuir waves and their influence on the surrounding plasma. One of the four flights flown in 1982 resulted in important measurements of the HF wave field strength, electron and ion temperatures, and suprathermal electrons excited by the heater (Rose et al., 1985), but the EISCAT radar was, unfortunately, not operational during this flight. A special modification to one of the antenna arrays had to be made in order for a rocket to be able to fly through the heated region, as rockets could not be flown over the Norwegian mainland. Phase delay coaxial feed lines were constructed for the mid-frequency Array 2 such that the heater beam could be tilted 13∘ westwards towards the planned rocket apogee, in addition to a tilt of 7.5∘ northwards achieved by normal phasing of the transmitters. These lines could be switched in and out within a few minutes, in the same way that the transmitters could be switched between different antenna arrays. This westward tilting ability was also used after the rocket campaigns for some satellite radio beacon experiments, but the coaxial lines of this phase shifter were later removed to reduce maintenance. However, the remaining switches and control hardware may still have a useful future, as will be mentioned below.
The most important results from the first phase of operation of the Heating facility, led by researchers from MPAe, have been summarized in Stubbe (1996).
The three antenna arrays were all used depending on which frequency was optimum for the particular science goal. Many experiments in which plasma instabilities were excited required pump frequencies near the O-mode critical frequency in the F layer, which has a daytime maximum that varies with solar radiation and the solar cycle. Other experiments that rely on maximum ohmic heating of the lower ionosphere are best with lower frequencies. Thus, Array 1, the lowest-frequency and largest array, was used mostly for VLF/ULF (ultra-low-frequency) modulation and other D region experiments. On 25 October 1985, extremely high winds during a storm seriously damaged about 75 % of the 36 antenna towers in Array 1, leaving all of the wooden masts intact. None of the towers in the other two arrays were damaged. The aluminium towers in all three arrays were of identical construction, differing only with respect to height: 12 m in Array 3, 16 m in Array 2, and 23 m in Array 1. They were anchored only at the top and bottom with no guy wires in between, which was probably the reason for the failure: the Array-1 towers were too tall and, hence, not rigid enough such that they bent under the force of the wind.
Rather than rebuild the array in its original form, it was decided that the lower-frequency band could be dispensed with, as VLF/ULF wave generation experiments could also be done at higher frequencies, and Landau damping of the Langmuir waves generated by parametric instabilities at these lower frequencies would be too high to make them interesting. On the other hand, one lost the opportunity to examine effects at the second gyro-harmonic, and one also lost flexibility with respect to the frequency choice near solar minimum, during which time the critical frequencies are often very low. It was decided to add 120 wooden masts of 12 m height to the existing 23 m high wooden masts and to install 144 crossed full-wave dipole antennas in a 12×12 configuration for the 5.5–8 MHz frequency band, within the same area as the 6×6 original low-frequency antennas (see Fig. 2). This gave the array a gain of 30 dBi, which corresponds to an ERP of 1200 MW compared with the 24 dBi of Array 3; thus, Array 1 was sometimes called the super heater. The resulting beam was, therefore, narrower than that of the other two arrays, and it could not be tilted as far off zenith. Experiments with the rebuilt array started in 1990 and continued under MPAe leadership until the transfer to EISCAT in January 1993. Although no new phenomena were discovered with the higher-gain array, the higher power density proved useful for many experiments, especially in the D region or mesosphere as later experiments were to show. A more detailed description of the HF facility, as it was then, is given in Rietveld et al. (1993).
After nearly 10 years of successful discoveries and exploration, it was decided that MPAe would end Project Heating. This was suggested by Ian Axford, as the emphasis of research at the institute was increasingly moving towards space-based instrumentation, and ionospheric research activity was decreasing. This was in line with a general policy within the Max Planck Society to fund research projects only for a limited time and to start new ones. A transfer of the facility to EISCAT Scientific Association was offered, and after a scientific case (Robinson et al., 1989) was prepared by a group of interested scientists led by Prof. Terry Robinson, the facility was formally transferred to EISCAT in January 1993. The first author, who had been employed at EISCAT since 1987, became responsible for running the facility from 1993 until December 2020, and, initially, two engineers were dedicated with the operation and maintenance of the Heating division of EISCAT. Gradually, the staff at Ramfjordmoen shared the tasks necessary to operate the IS radars and Heating as required by the changing user demands of the different facilities. For example, much effort was spent on building the EISCAT Svalbard Radar (ESR) in the early to mid 1990s which diverted scientific interest and operations to the polar cap region.
The transfer to EISCAT resulted in a larger user group continuing heating experiments that nominally used 200 h of heater time per year but which varied between 100 and 300 h depending partly on the solar cycle. Fewer experiments were possible when the F region critical frequency was low during solar minimum. Most experiments were in conjunction with the IS radar as the major diagnostic instrument (in many cases) or as one of several diagnostics. Improvements in diagnostic instrumentation; the deployment of new instruments; and improved radar coding, modulation, and data storage associated with advances in computing technology led to new discoveries that were impossible or difficult to achieve in the first decade of operation. Some examples are (1) the discovery that heating the lower ionosphere can weaken or suppress polar mesospheric summer echoes (PMSE) observed by the VHF (224 MHz) radar (Chilson et al., 2000) and (2) the production of light emission from the heated ionosphere (Brändström et al., 1999). These two very different areas of research, namely study of the mesospheric dusty plasma and energetic electron acceleration, have remained as major topics of research up to the present time.
A technique developed in the former Soviet Union that uses only the powerful HF waves to measure ionospheric and atmospheric parameters by the production of artificial periodic irregularities (API) (Belikovich et al., 2002) was successfully applied in the auroral ionosphere for the first time by combining the Heating facility as a transmitter and the Dynasonde as a receiver (Rietveld et al., 1996b). Later, more sophisticated experiments by Vierinen et al. (2013) showed how this technique is particularly interesting and particularly promising for studies of the mesosphere.
The Heating facility remained essentially unchanged through the 1990s. An operating licence was obtained to allow frequency-stepping experiments around harmonics of the gyro-frequency. There was an upgrade of the computer from the original Commodore PET to a Microsoft Windows-based personal computer system in 1999, when the original BASIC control program was converted to Hewlett Packard BASIC, and the more modern computer allowed RF synthesizer and transmitted HF parameters to be stored in a digital log. Previously, the transmitter settings had been recorded largely in a hand-written log book. There were minor changes made to some of the control system, such as a programmable step change in the control grid bias voltage during long (>ca.1 s) “RF off” intervals such that the quiescent current in the transmitter tubes dropped from about 6 to 1 A (at 10 kV in each of 12 transmitters!) to save on electricity power consumption. One saves most on power consumption when the high voltage is switched off so that no quiescent current flows through the tube, but this had to be done manually by pushing 12 buttons that actuated 12 large relays, something that is undesirable for non-transmitting intervals of a few seconds, tens of seconds, or a few minutes, which are rather commonly used modulation periods. The cost of electric power for Heating operation, especially when experiments required Heating and the VHF and UHF radars, was a major economic concern in the 1990s at a time when there were some EISCAT associates who were advocating for the closure of the Heating facility to save money.
Around 2005, plans were made to upgrade the synthesizers to direct digital synthesis (DDS) and the associated computer control to a unix-based system. Apart from replacing ageing hardware, a major motivation was to allow fast frequency changes of the HF pump wave which were increasingly requested in order to examine the ionospheric response to HF pumping at and near harmonics of the ionospheric gyro-frequency. Previously, frequency changes required a several-minute-long tuning and phasing procedure under computer control, as the HP synthesizers started with a random phase value for any frequency change. The digital system would allow setting of phases to any desired values practically instantaneously. The final system, which was taken into regular use in 2009, used some hardware and much software that the EISCAT IS radars had implemented in the mid-1990s when the ESR was built. This upgrade was a major effort involving the expertise of EISCAT staff from Tromsø as well as the other two mainland sites, the EISCAT headquarters in Kiruna, Sweden, and Sodankylä in Finland. This upgrade and further improvements to the Heating system are described in Rietveld et al. (2016). From 2012, even more functionality has been developed such that the status of many transmitter and array parameters that were only indicated by lights or controllable by buttons are now monitored and set by computer. Figure 6 shows the console in the control room before the upgrade. The main difference after the upgrade is the replacement of the 12 original synthesizers in the central part with large computer screens that are now used to control and monitor the facility's operation and observe some scientific results in real time.
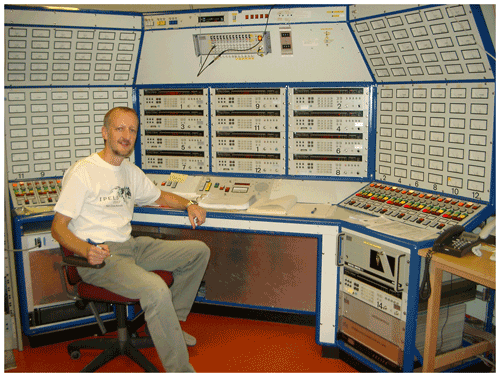
Figure 6The Heating facility console in the control room as it was in 2007, with the first author at the controls. Six columns of meters, lights, and push buttons on each side show the status and are used to control each of the 12 transmitters. The 12 commercial RF synthesizers in the middle of the console have been replaced by digital synthesizers in the transmitter hall, and the space is now filled with large computer screens. (Photo credit: Michael T. Rietveld)
In 2013, a modification was made to the coaxial switches that fed Array 3 to allow receivers to be connected to that array. The motivation for this was to try and receive magnetospheric echoes, for example, from ion acoustic turbulence excited by auroral processes such as has been observed by the VHF and UHF IS radars (Rietveld et al., 1996a). Previously, related experiments had been tried using Heating as a transmitter and the large HF radio telescope, UTR2, in Ukraine as a receiver, although without results. As the modified Array 1 and Array 3 cover the same frequency range, one could transmit on Array 1 and receive on Array 3, albeit with different antenna gains and, hence, beam widths. The first version of a receiver connected to Array 3 for radar work is described in Rietveld et al. (2016), where fixed-length phasing cables were used to combine the signals from the six rows of orthogonal antennas into two receiver channels. Since 2017, each individual row of antennas is connected to a digital receiver allowing beam forming of the received signal in the north–south plane. This receiving system seems to work well for mesospheric echoes, but echoes from the magnetosphere have not been detected to date. Using Array 3 as a receiving antenna has some weaknesses, such as the aluminium connectors in the feeder lines where an oxide layer may adversely affect weak radio signals, although this layer is burnt through by the powerful radio wave on transmission for which it was designed.
The Heating facility was only intended to operate for a limited time of about 10 years; thus, 40 years after construction, it was inevitable that some parts of the system had aged to a critical point or that spare parts had become unobtainable. One key component is the transmitting tube in each of the 12 power amplifiers. The original tube was no longer produced after 1980, but a good number of spares allowed operation at near-full-power level until recently. Very few tubes failed completely, but after about 12000 h of filament-on time over the lifetime of Heating, several were slowly delivering less power. A few tubes were sent to firms in the USA to be rebuilt, but the success rate was poor. In searching for an alternative tube that required minimal modification to the transmitters, it was found that the RS2054SK tetrode was almost compatible with the existing transmitter, and this tube type was still manufactured in Europe and in China. Although it was a drop-in replacement in the tube socket, the new tube required a different filament voltage and a slow ramping up and down of the voltage so that several modifications to the transmitter had to be made. In 2018, the first of the new tubes entered operation, and two transmitters presently use the new tubes with a third ready to be similarly modified.
Another ageing problem that first appeared around 2008 was in the modified Array 1. The feed cables to the antennas in this array were different from the original design in that, instead of towers made of aluminium coaxial cable feeding each antenna, commercial twin-wire flexible cable was used. Starting in 2008, an increasing number of the 288 feed points at the antenna failed during transmission through burning insulation and fire, often resulting in the collapse of the whole feed point and antenna to the ground and sometimes resulting in a dramatic grass fire. The cause of this failure was a mystery for a long time, and a total of 17 feed cables and the centre parts of the antennas had required laborious repairs by 2017. In 2015, the cause of this failure was found to be metal fatigue in the flexible twin-wire braided-copper cable where it was anchored to the fixed centre of the antenna, with the rest of the feed cable from near the ground having been free to move slightly in the wind for about 20 years. Many of the anchoring guy ropes which should have minimized movement of the cable in the wind had broken and were not repaired. The wind-induced movement of the cable caused many of the braids to break such that the cross-sectional area of the cable was reduced to the point that the high-power RF caused overheating or arcing across the final break, resulting in burning of the insulation. The solution was to bypass the anchor point with a short piece of wire crimped to the feed wires on each of the 144 antenna centres on the 12 m tall masts, a job which took several summer seasons and required EISCAT to buy a lift to safely implement the repairs. It is hoped that this solution is robust enough for the remaining lifetime of the Heating facility. Figure 7 shows the repair work in Array 1, where the different feed cables (compared with those in Fig. 3) are clearly visible.
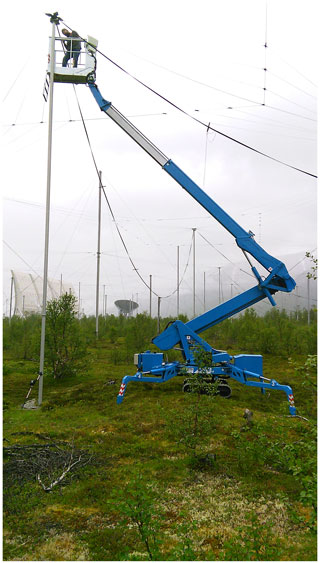
Figure 7Repair work to one of the 12 m high antennas out of 144 such antennas in Array 1 to bypass existing and potential weak points in the twin-feed cable connection to the antenna centre. The original 23 m wooden masts are visible in the background. Note the different support mast and the different feed lines compared with the original design as used in Array 2 and Array 3, shown in Fig. 3. (Photo credit: Michael T. Rietveld)
The main hardware of Heating, the transmitters, feed lines, and antennas, have remained essentially unchanged since 1990, apart from the computer control and RF synthesizer upgrades described above. The user community has changed with time, with some users and groups changing field, but there are also new users entering the field. With the closure of other facilities like the HIPAS (HIgh Power Auroral Stimulation) Observatory in Alaska (Wong et al., 1990) and SPEAR (Space Plasma Exploration by Active Radar) on Svalbard (Robinson et al., 2006) as well as the hopefully temporary closure of the Arecibo heating facility, the only sites with working HF ionospheric heating facilities are HAARP (High-frequency Active Auroral Research Program) in Alaska (Pedersen and Carlson, 2001) and Sura in Russia (Belikovich et al., 2007). On 1 December 2020, after 57 years of usage, Arecibo Observatory's 900 t platform containing transmit–receive feeds fell ∼150 m and crashed into the 305 m diameter reflector dish. This halted IS radar, HF heating, planetary radar, and radio astronomy observations at the observatory. Full or partial recovery plans are currently under consideration by the US National Science Foundation. Complete decommissioning appears unlikely, and a modest HF facility is currently being constructed at Arecibo to keep HF heating research moving forward. However, none of these other installations will have an IS radar as a diagnostic instrument in the foreseeable future, which makes the Tromsø HF heater a unique and valuable facility for the world.
Groups from all the EISCAT Associates have been regular users of the Heating facility. In recent years, researchers from China – the China Research Institute of Radio Wave Propagation (CRIRP) became an EISCAT Associate in 2007 – have become important regular users. A large international community of scientists have been able to use the Tromsø HF facility, especially in the last decade, as non-EISCAT Associates could either buy time or apply for a limited number of free hours on either the IS radar, heater, or both via a peer-review programme. An excellent example of fruitful scientific results from heating experiments by a non-EISCAT Associate is a 25-year collaboration with a group from the Russian Arctic and Antarctic Research Institute (Blagoveshchenskaya et al., 2020). Other long-term users were researchers from the Polar Geophysical Institute, in Murmansk, Russia, and from the Institute of Radio Astronomy in Ukraine. A description of the various scientific results that have been obtained from the Heating facility is beyond the scope of this paper. The number of accumulated publications from the Tromsø heating facility amounts to more than 490, and these papers are listed on the EISCAT publications web page (https://eiscat.se/scientist/publications/heating-publications/, last access: 3 March 2022). Streltsov et al. (2018) discuss many of the physical problems that are topics of present and future research in the field of active experiments using high-power radio waves. Some of the interesting phenomena to explore are narrowband SEE (stimulated Brillouin scatter), artificial ionization, unexplained X-mode effects, and the irregularities postulated to explain wide-altitude ion line enhancements sometimes known by the acronym WAILES (Rietveld and Senior, 2020).
The Tromsø heating facility has not been overly troubled by adverse publicity or conspiracy theories. The experiments conducted at the Tromsø facility were always open, and all publications resulting from it appear in the open literature; we believe this is true for nearly all of the experiments performed at the other facilities as well. Nevertheless, over the years, there have been exaggerated and false claims and conspiracy theories made about some of the experiments that are possible with heating facilities like HAARP in Alaska and Heating in Tromsø.
The site in Ramfjordmoen is about to undergo a major change when the EISCAT UHF and VHF radars are decommissioned and EISCAT_3D, the next-generation IS radar (McCrea et al., 2015), starts operation, with the core site in nearby Skibotn. Since the retirement of the first author, the Heating facility has been led and run by Erik Varberg. The Heating facility is planned to remain in operation for experiments with the new radar which will offer unprecedented insights into HF-induced phenomena. The improved spatial resolution and the ability to quickly steer the beam of the new radar electronically or to have multiple beams should help probe the horizontal spatial properties of HF-induced irregularities. There is one disadvantage to not having the HF facility and the radar co-located, namely the radar cannot probe in the field-aligned direction along the heater beam in the F region. This problem may be overcome by resurrecting, in a slightly modified form, the east–west tilting hardware built for the HERO rocket campaign in the 1980s mentioned earlier. Most of the switching hardware still exists, but new aluminium coaxial phasing cables would need to be made and installed. The possibility of building a new heater nearer Skibotn is also being investigated.
No data sets were used in this paper. All citations appear in the reference list.
MTR wrote most of the paper, and PS provided additions and corrections.
The contact author has declared that neither they nor their co-authors have any competing interests.
Publisher's note: Copernicus Publications remains neutral with regard to jurisdictional claims in published maps and institutional affiliations.
In addition to the people mentioned in this paper, we thank the large number of unnamed staff from MPAe and EISCAT as well as other collaborators, who helped build, operate, and maintain this remarkable scientific facility. EISCAT is an international scientific association presently supported by research organizations in China (CRIRP), Finland (SA), Japan (NIPR and STEL), Norway (NFR), Sweden (VR), and the United Kingdom (NERC).
This paper was edited by Kristian Schlegel and reviewed by two anonymous referees.
Belikovich, V., Benediktov, E. A., Tolmacheva, A. V., and Bakhmet'eva, N. V.: Ionospheric research by means of artificial periodic irregularities, ISBN 3-936586-03-9, Göttingen, Copernicus GmbH, 2002.
Belikovich, V. V., Grach, S. M., Karashtin, A. N., Kotik, D. S., and Tokarev, Yu. V.: The SURA facility: study of the atmosphere and space, Radiophys. Quantum El., 50, 7, 497–526, 2007.
Blagoveshchenskaya, N. F.: Perturbing the High-Latitude Upper Ionosphere (F Region) with Powerful HF Radio Waves: A 25-Year Collaboration with EISCAT, Radio Science Bulletin, 373, 40–55, 2020.
Brändström, B. U. E., Leyser, T. B., Steen, Å., Rietveld, M. T., Gustavsson, B., Aso, T., and Ejiri, M.: Unambiguous evidence of HF pump-enhanced airglow at auroral latitudes, Geophys. Res. Lett., 26, 3561–3564, 1999.
Chilson, P. B., Belova, E., Rietveld, M. T., Kirkwood, S., and Hoppe, U.-P.: First artificially induced modulation of PMSE using the EISCAT heating facility, Geophys. Res. Lett., 27, 3801-3804, 2000.
Czechowsky and Rüster: 60 Jahre Forschung in Lindau (in German), Copernicus Publications, Göttingen, ISBN 979-3-936586-65-7, 2007.
Fejer, J. A. and Kopka, H.: The effect of Plasma instabilities on the ionospherically reflected wave from a high power transmitter, J. Geophys. Res., 86, 5746–5750, 1981.
Haerendel, G.: History of EISCAT – Part 4: On the German contribution to the early years of EISCAT, Hist. Geo Space. Sci., 7, 67–72, https://doi.org/10.5194/hgss-7-67-2016, 2016.
Holt, O.: History of EISCAT – Part 3: The early history of EISCAT in Norway, Hist. Geo Space. Sci., 3, 47–52, https://doi.org/10.5194/hgss-3-47-2012, 2012.
Kopka, H., Stubbe, P., and Zwick, R.: On the ionospheric modification experiment projected at MPI Lindau: Practical realization, AGARD Conference Proceedings, 192, 10.1–10.7, 1976.
Leyser, T. B.: Stimulated electromagnetic emissions by high-frequency electromagnetic pumping of the ionospheric plasma, Space Sci. Rev., 98, 223–328, 2001.
McCrea, I., Aikio, A., Alfonsi, L., Belova, E., Buchert, S., Clilverd, M., Engler, N., Gustavsson, B., Heinselman, C., Kero, J., Kosch, M., Lamy, H., Leyser, T., Ogawa, Y., Oksavik, K., Pellinen-Wannberg, A., Pitout, F., Rapp, M., Stanislawska, I., and Vierinen, J.: The science case for the EISCAT_3D radar, Progress in Earth and Planetary Science, 2, 21, https://doi.org/10.1186/s40645-015-0051-8, 2015.
Pedersen, T. T. and Carlson, H. C.: First observations of HF heater produced airglow at the High Frequency Active Auroral Research Program facility: thermal excitation and spatial structuring, Radio Sci., 36, 1013–1026, https://doi.org/10.1029/2000RS002399, 2001.
Rietveld, M. T. and Senior, A.: Ducting of incoherent scatter radar waves by field-aligned irregularities, Ann. Geophys., 38, 1101–1113, https://doi.org/10.5194/angeo-38-1101-2020, 2020.
Rietveld, M. T., Kohl, H., Kopka, H., and Stubbe, P.: Introduction to ionospheric heating at Tromsø-I. Experimental overview, J. Atmos. Terr. Phys., 55, 577–599, 1993.
Rietveld, M. T., Collis, P. N., van Eyken, A. P., and Løvhaug, U. P.: Coherent echoes during EISCAT UHF Common Programmes, J. Atmos. Terr. Phys., 58, 161–174, 1996a.
Rietveld, M. T., Turunen, E., Matveinen, H., Goncharov, N. P., and Pollari, P.: Artificial Periodic Irregularities in the Auroral Ionosphere, Ann. Geophys., 14, 1437–1453, 1996b.
Rietveld, M. T., Wright, J. W., Zabotin, N., and Pitteway, M. L. V.: The Tromsø Dynasonde, Polar Sci., 2, 55–71, https://doi.org/10.1016/j.polar.2008.02.001 2008.
Rietveld, M. T., Senior, A., Markkanen, J., and Westman, A.: New capabilities of the upgraded EISCAT high-power HF facility, Radio Sci., 51, 1533–1546, https://doi.org/10.1002/2016RS006093, 2016.
Robinson, T. R., Stubbe, P., Thidé, B., Rietveld, M., and Mjølhus, E.: A New Heating Facility for EISCAT: Scientific Case, EISCAT Technical Report, https://eiscat.se/wp-content/uploads/2021/05/Robinson_report_1989.pdf (last access: 3 March 2022), 1989.
Robinson, T. R., Yeoman, T. K., Dhillon, R. S., Lester, M., Thomas, E. C., Thornhill, J. D., Wright, D. M., van Eyken, A. P., and McCrea, I.: First observations of SPEAR induced artificial backscatter from CUTLASS and the EISCAT Svalbard radar, Ann. Geophys., 24, 291–309, 2006.
Rose, G., Grandal, B., Neske, E., Ott, W., Spenner, K., Holtet, J., Måseide, K., and Trøim, J.: Experimental Results From the HERO Project: In Situ Measurements of Ionospheric Modifications Using Sounding Rockets, J. Geophys. Res., 90, 2851–2860, 1985.
Senior, A., Rietveld, M. T., Honary, F., Singer, W., and Kosch, M. J.: Measurements and modelling of cosmic noise absorption changes due to radio heating of the D-region ionosphere, J. Geophys. Res., 116, A04310, https://doi.org/10.1029/2010JA016189, 2011.
Streltsov, A. V., Berthelier, J.-J., Chernyshov, A. A., Frolov, V. L., Honary, F., Kosch, M. J., McCoy, R. P., Mishin, E. V., and Rietveld, M. T.: Past, Present and Future of Active Radio Frequency Experiments in Space, Space Sci. Rev., 214, 118, https://doi.org/10.1007/s11214-018-0549-7 2018.
Stubbe, P.: Review of ionospheric modification experiments at Tromsø, J. Atmos. Terr. Phys., 58, 349–368, 1996.
Stubbe, P., Kopka, H., and Rose, G.: Rocket experiments in conjunction with the ionospheric modification experiment in northern Norway, edited by: Halvorsen, T. and Battrich, B., Proceedings of Esrange Symposium, Ajaccio, 24–29 April, ESA SP-135, 107–111, 1978.
Stubbe, P., Kopka, H., Brekke, A., Hansen, T., Holt, O., Dowden, R. L., Jones, T. B., Robinson, T. R., Lotz, H.-J., and Watermann, J.: First results from the Tromsø ionospheric modification facility, AGARD Conference Proceedings, 295, 16.1–16.9, 1980.
Stubbe, P., Kopka, H., Lauche, H., Rietveld, M. T., Brekke, A., Holt, O., Jones, T. B., Robinson, T., Hedberg, Å., Thidé, B., Crochet, B., and Lotz, H.-J.: Ionospheric modification experiments in northern Scandinavia, J. Atmos. Terr. Phys., 44, 1025–1041, 1982.
Stubbe, P., Kopka, H., Thidé, B., and Derblom, H.: Stimulated electromagnetic emission: A new technique to study the parametric decay instability in the ionosphere, J. Geophys. Res., 89, 7523–7536, 1984.
Stubbe, P., Kopka, H., Rietveld, M. T., Frey, A., Høeg, P., Kohl, H., Nielsen, E., Rose, G., LaHoz, C., Barr, R., Derblom, H., Hedberg, Å., Thidé, B., Jones, T. B., Robinson, T., Brekke, A., Hansen, T., and Holt, O.: Ionospheric modification experiments with the Tromsø heating facility, J. Atmos. Terr. Phys., 47, 1151–1163, 1985.
Tellegen, B. D. H.: Interaction between radio waves, Nature, 131, 840, https://doi.org/10.1038/131840a0, 1933.
Thidé, B., Kopka, H., and Stubbe, P.: Observations of stimulated scattering of a strong high-frequency wave in the ionosphere, Phys. Rev., Lett., 49, 1561–1564, 1982.
Utlaut, W. F.: Foreword to special issue on ionospheric modification, Radio Science, special issue, 9, 881–1090, 1974.
Vierinen, J., Kero, A., and Rietveld, M. T.: High latitude artificial periodic irregularity observations with the upgraded EISCAT heating facility, J. Atmos. Sol.-Terr. Phy., 105–106, 253–261, 2013.
Wannberg, G.: History of EISCAT – Part 5: Operation and development of the system during the first 2 decades, Hist. Geo Space. Sci., 13, 1–21, https://doi.org/10.5194/hgss-13-1-2022, 2022.
Wong, A. Y., Carroll, J., Dickman, R., Harrison, W., Huhn, W., Lure, B., McCarrick, M., Santoru, J., Schock, C., Wong, G., and Wuerker, R. F.: High-power radiating facility at the HIPAS Observatory, Radio Sci., 25, 1269–1282, 1990.
- Abstract
- Introduction
- Background and conception
- Funding, construction and inauguration
- The first decade of discoveries
- A storm and antenna array reconfiguration
- Transfer to EISCAT and user expansion
- Present status and future
- Data availability
- Author contributions
- Competing interests
- Disclaimer
- Acknowledgements
- Review statement
- References
- Abstract
- Introduction
- Background and conception
- Funding, construction and inauguration
- The first decade of discoveries
- A storm and antenna array reconfiguration
- Transfer to EISCAT and user expansion
- Present status and future
- Data availability
- Author contributions
- Competing interests
- Disclaimer
- Acknowledgements
- Review statement
- References