the Creative Commons Attribution 4.0 License.
the Creative Commons Attribution 4.0 License.
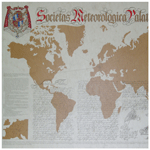
The early meteorological network of the Societas Meteorologica Palatina (1781–1792): foundation, organization, and reception
Peter Winkler
The Societas Meteorologica Palatina arranged the first international meteorological network in a modern sense, being in operation between 1781 and 1792 during the last period of enlightenment. A total of 39 stations contributed observations. The original aim was to investigate influences of the moon and planets on the atmosphere. Instruments were provided free of charge; a physically very advanced instruction guaranteed reliable observational results, and the data collected at 3 different hours per day were printed at high cost in the Ephemerides Societatis Meteorologicae Palatinae (denoted Ephemerides hereafter) of Mannheim. This wealth of data has become a famous treasure trove for scientists and has been used later very often for climatic studies, for climatic comparisons of different locations in textbooks, for overcoming wrong but generally accepted or even outdated (e.g. scholastic) views; for finding new explanations for meteorological phenomena, and for studying extremes of meteorological parameters. Even in modern times, the data were evaluated and used to reconstruct historical weather maps. Although, meanwhile, some problems of the historical instruments have been recognized, most of the conclusions are still basically correct. The data were also used for verifying geomagnetic models or proxy data from tree-ring analysis. This network stimulated many scholars for special meteorological studies, and it was attractive for new stations to join the network. The early death of the meteorological secretary Johann Jakob Hemmer and the Napoleonic Wars brought about the end of the project. Nevertheless, many of the stations continued the observations using the available instruments.
- Article
(12423 KB) - Full-text XML
- BibTeX
- EndNote
Galileo's famous aphorism “Measure what can be measured, and make measurable what cannot be” stimulated scholars as well as instrument makers in the Age of Enlightenment to a new approach in analysing and interpreting natural phenomena, including the atmosphere.1 The philosopher Christian Wolff, who had a strong spiritual influence on the Enlightenment in Europe, reformulated Galileo's aphorism in his Mathematisches Lexikon (Wolff, 1716, Sp. 863f.), without mentioning Galilei: “Ist eine Wissenschaft alles auszumessen, was sich ausmessen lässt” [There is a science of measuring all what can be measured]. When meteorological instruments became available, regular meteorological observations were started by interested professors at some universities or at scientific academies. The latter even included the intention for meteorological observations in their statutes. However, production and calibration of meteorological instruments were still a demanding task, and no general agreement existed on how observations should be performed. Each scholar and each institution set their own rules, and at best, data exchange was arranged on a private basis. In this situation, the realization of an international meteorological society by the Academy of Sciences in Mannheim was a very attractive, promising, and eventually successful project.
Countless publications have appeared already about the Societas Meteorologica Palatina, the most informative of which are Kistner (1930), Cappel (1980b), Cassidy (1985), Kington (1988), and Frångsmyr et al. (1990). All emphasize the following as the most important achievements:
-
free provision of uniformly manufactured and calibrated instruments;
-
instruction for installing instruments and doing observations;
-
invitation of institutions rather than private persons as observers to ensure continuity;
-
uniform observation times at 07:00, 14:00, and 21:00 LT (local time), the so-called Mannheim hours;
-
uniform registers, to be sent in by diplomatic mail;
-
printing the extenso observations in the society's Ephemerides.
In the past, several attempts were undertaken to set up a meteorological network: 1654–1667 by the Accademia del Cimento in Florence (10 stations), 1662 by the Royal Society in London (number of stations unknown), and 1776 by the Royal Society of Medicine in Paris (32 to 65 stations). They were not nearly as successful as the Academy of Sciences in Mannheim (e.g. Libri, 1830; Hellmann, 1914; Rigby, 1965; Fleming, 2002; Camuffo, 2000).
The progress achieved by the Societas Meteorologica Palatina consisted of the instruments which were manufactured under the supervision of a physicist and uniformly calibrated. This successful example has demonstrated the feasibility of international cooperation in meteorology, which later served as a model when new measuring networks were set up. This meteorological network was created due to the personal engagement and organizational talent of its secretary, Johann Jakob Hemmer. The collected data have been re-evaluated since then for many applications. One should be aware that the local view of weather determined the imagination of the meteorological development and that farmers rules were the only known means for forecasting weather.
The purpose of the present work is to report on less-known or even overlooked aspects (see below) that played a role in the implementation of the Societas Meteorologica Palatina, including the preceding mental development, instrumental deficiencies, and data quality. Moreover, I will bring some examples for the broad reception of the datasets by scientists in many fields of knowledge.
During the last phase of enlightenment, the usefulness of scientific efforts played a predominant role under regents who had to decide which project should be funded. Two examples may illustrate this point. Firstly, lightning rods had proven to protect buildings from ignition, and physicists had understood the mode of functioning. Therefore, decrees were enacted to install lightning rods on all public buildings (Mayr, 1788, p. 674, regulation from 23 December 1784). The second example is the drainage of moors in order to cultivate barren land. The governments expected that the productivity of their country would rise without, in that case, having knowledge of the ecological function of moors (Mayr, 1788, p. 663, regulation from 15 May 1783).
In meteorology of the 18th century, the gravity pull of the moon on the atmosphere was known and assessed to be 850 times larger than the oceanic tide. While Daniel Bernoulli (1700–1782) had recognized the compressibility of the atmosphere and refrained from calculations of atmospheric tidal calculations (Bernoulli, 1987, p. 270), Kratzenstein (1723–1795) considered the atmosphere as incompressible (Fig. 1) and expected a large lunar tide (Kratzenstein, 1747). The latter simple hypothesis had a better reception among scholars than unsolved mathematical problems.
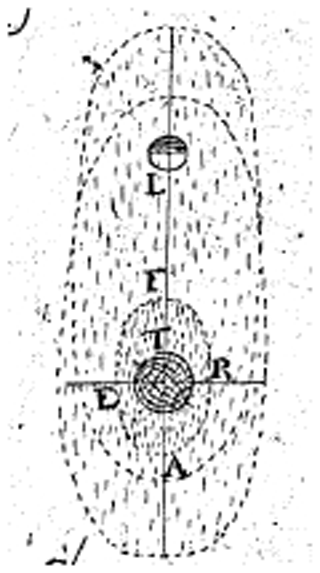
Figure 1Gravitational deformation of the earth's atmosphere A by the moon L (Kratzenstein, 1747: table in the appendix, Fig. 1).
Scholars argued that in the atmosphere predictable pressure oscillations would be induced by the moon and could be measured. A meteorological observation network would enable scholars to analyse such regular waves and allow for the prediction of weather, once long enough time series of meteorological parameters were available. Agriculture would take profit from such predictions, convincing Elector Karl Theodor from Palatine (denoted Elector hereafter) to invest money in such an important project. A rapid result was expected, whereas any promotion of basic science was not a governmental goal. In continuation of the mechanics of Newton, the generally prevailing view was that the world is functioning like a machine.
2.1 Foundation of the Societas Meteorologica Palatina
The foundation of a meteorological society in Mannheim was the result of a special constellation of several persons (Fig. 2; Kistner, 1930; von Stengel and Ebersold, 1993): the privy counsellor and minister for education Georg von Stengel (1721–1798) of Elector2 Karl Theodor (1724–1799) had started private meteorological observations three times per day in 1758, and his son Stephan (1750–1822) helped him in cases when he was tied up with business. Karl Theodor was personally interested in physics, and he made weather observations in his castles and during journeys (Kistner, 1930). He had studied law at Leiden University (the Netherlands) and probably might have come into contact with the widely known professors s' Gravesande or Musschenbroek, who were teaching physics. Stephan von Stengel was an illegitimate child of the Elector, who followed him to Munich in 1779 as secretary, when Karl Theodor became successor of the childless Elector Max III of Bavaria at the end of 1777.
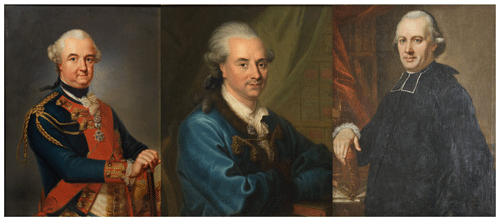
Figure 2(a) Portrait of Karl Theodor 1724–1799 (source: Bavarian National Museum, Munich, Inv. no. L 2009/40.1; photo: Bastian Krack); (b) portrait of Stephan von Stengel 1750–1822 (source: Reiss-Engelhorn-Museen, Mannheim, Inv. no. O 333; photo: Jean Christen); (c) Johann Jakob Hemmer 1733–1790 (source: painting privately owned, owner not publicly known).
Karl Theodor was interested in physics and had installed a collection of physical instruments, for which he hired the court chaplain Johann Jakob Hemmer (1733–1790) as custodian. Karl Theodor often spent several hours in the physical cabinet, learning physical experiments arranged by Hemmer. He had recognized the progress which could be achieved in explaining natural phenomena by means of experiments instead of magic explanations or the antiquated physics of the Jesuits, being based on their scholastic Aristotelian teaching methods or Descartes' meteorological theses (see Sect. 2.2).
After the move of Karl Theodor to Munich, Stephan von Stengel started meteorological observations and motivated a military engineer in Mannheim to do the same at fixed times. They exchanged their data every 10 d, and from Hemmer von Stengel obtained information on atmospheric electricity. Stephan von Stengel prepared comparative tables which he regularly presented to Karl Theodor, and he was able to arouse his interest. Inspired by the plan of Lorenz Böckmann (1741–1802) in Karlsruhe in the neighbouring state of Baden to implement a meteorological network of 16 stations in 1779 (Cassidy, 1985; Lüdecke, 2010), Hemmer as well as von Stengel discussed the possibility to implement another meteorological network in Palatine or even in Europe (Kistner, 1930; von Stengel and Ebersold, 1993).
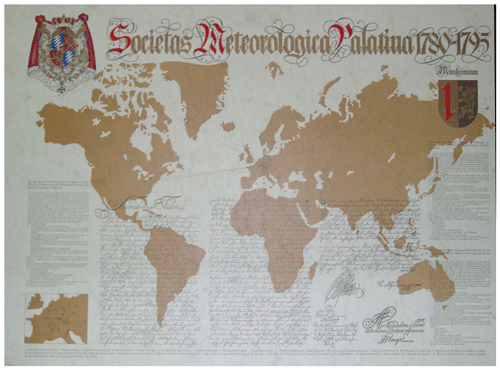
Figure 3Collage containing a copy of the foundation charter in the library of the German Weather Service, most probably compiled on the occasion of the 200-year anniversary of the Societas Meteorologica Palatina in 1980 (Symposium, Mannheim, 13–15 October 1980).
In Baden, progress was hampered due to a delay in manufacturing of meteorological instruments, which eventually resulted in the failure of the project. However, Stephan von Stengel, being a good organizer of statesmanlike projects and having a good overview on meteorological observations already running at other places, succeeded in motivating Elector Karl Theodor to found a third meteorological class at the Academy of Sciences in Mannheim in 1780, and nominating Hemmer as the secretary (Fig. 3).
The scientific goal was to analyse periodic developments in the course of weather induced in the atmosphere by the gravitation of the moon (see Sect. 2.2).
Stephan von Stengel and Hemmer had postulated that “harmonizing” instruments was necessary in order for the meteorological network to become successful. Hemmer sent relevant expert reports to von Stengel in Munich. Obviously, both knew of the difficulties in manufacturing and calibrating meteorological instruments. Karl Theodor could be convinced that, for the success of such a project, standard calibration instruments should be implemented in the entire network. Stephan von Stengel, after consultations with his father Georg von Stengel and Hemmer, worked out the document of the foundation charter which was signed by the Elector on 15 September 1780 in Munich, who financed the instruments from his cabinet treasury.
To understand the enormous efforts and outstanding success of this initiative, it is important to remember that doing science at that time was not a matter of course. Academies had to be protected by the country's regent, and they had to achieve to be exempt from censorship. Although the Jesuit order was abolished, many former members remained in the teaching profession and tried to hinder academic research (Müller, 1986, 1993). Their method of teaching at the universities and grammar schools remained unchanged on the Ratio Studiorum introduced in 1599, and their influence persisted even after the abolition of their order in 1773. In addition, Karl Theodor's confessor was an ex-Jesuit who was not well disposed toward the academy but who had a significant influence on his decisions. Many progressive courtiers and members of the government or administrative officials, even if they were educated by Jesuits, became academic members although they rarely contributed to research. Their main task was to support and enable research activities, ward off hostile and envious currents, and eliminate resistances since other parts of the society could not see a point to “useless” research. Thus, the academies in Mannheim and in Munich had to act wisely and cleverly at the time of feudalism.
Hemmer presented a speech to the Academy of Sciences on 21 October 1780.3 He was a well-known scientist, who had published results of physical experiments, lightning rod installations, and application of electricity to heal human diseases. Thus, when Benjamin Thompson, the later Count Rumford, started his experiment in Mannheim to elucidate the existence of infrared heat radiation by drilling a cannon in order to demonstrate that phlogiston was not existing, Hemmer was invited as a critical observer and experienced experimentalist. If phlogiston should be responsible for the warming during drilling, the heating should cease when all phlogiston would have escaped. His drilling with a blunt drill was continued for many days but the cannon was as hot as at the beginning. They concluded that the heat was generated by friction and not by phlogiston (Meyer, 1806–1808). Hemmer's organizational capability was gravely missed after he had died.
2.2 The Influence of Lambert and Toaldo
Johann Heinrich Lambert (1728–1777) was a member of the Bavarian Academy of Sciences, and in a paper published in 1761 he developed a programmatic system for regular observations in agronomy, medicine, and meteorology (Lambert, 1761). We have no information on whether Georg von Stengel or Hemmer in Mannheim (or even Böckmann in Karlsruhe) had received knowledge of this paper, but we cannot exclude that they obtained a copy, since both academies exchanged information. Lambert recommended three observations per day and the use of the following instruments and measurements: a thermometer in the shadow and in the sun; barometer; atmospheric moisture measurement; evaporimeter; pluviometer; measurement of soil temperatures at several depths; river level; wind speed and direction; state of the heaven; cloud cover; meteorological events like fog, rain, thunderstorm, hail, and so on; state of soil (moist or dry); snow cover; colour of the heaven during sunrise and sunset; polar lights; sunshine duration; monthly minima and maxima of atmospheric pressure; and other meteorological parameters. Additionally, in a special column he recommended measuring the phases of the moon, solar elevation, and positions of planets in the zodiac circle. He also expected to be able to recognize any astronomical influences. In addition, a magnetic needle instrument should be observed to learn about changes in the geomagnetic field. In his “Exposé”, he recommended a worldwide observation network (Lambert, 1773). This is important, because at his time the local view on the weather was prevailing, and atmospheric pressure systems and their extension were unknown.
In 1762, Lambert calculated the astronomical calendar of the Bavarian Academy of Sciences, where he reported in an appendix “On the Meteorology” that analysis of atmospheric pressure data had shown only marginal effects by the moon (Lambert, 1762). As is often the case with science, his insight was ignored, and scholars tended to prove their own hypotheses: in this case, the hypothesis of a strong influence by the moon.
Lambert recommended to compare the wind direction with the average atmospheric pressure (Lambert, 1765). He was one of the first to argue that wind forms as a result of differences in atmospheric pressure and overcame the traditional explanation developed by Cartesius: winds can be compared with vapours which move from one place to another where they can expand with less effort (Descartes, 1637, p. 189f.). His experimental proof was the heating of water in a suffocated ball blowing out vapour from a hole in its wall.
Lambert's conclusion and recommendation was to investigate meteorology with the methods of astronomers. In this way, physical laws could be recognized and mathematically formulated. Once the main laws were found, one could study the deviations, identify the responsible processes, and be able to describe them mathematically. The mathematical formulas would be refined with time and would allow one to obtain methods for meteorological predictions.
Toaldo in Padua was a representative of scientists being convinced that astronomical influences on atmospheric pressure and the development of the weather would exist. His agrometeorological essay, which had won the prize of the academy in Montpellier in 1774, was published in Paris in October 1777 (Toaldo, 1777a) and translated in the same year into German (and other languages). At least three editions appeared. Toaldo had further developed earlier ideas of Duhamel (1623–1706) and Mairan (1678–1771). Also, Hemmer had received knowledge of Toaldo's paper, because early in 1779 he wrote to the Elector with the petition that the meteorological observations should be intensified with respect to agricultural requirements and be supplemented by observations on geomagnetism and atmospheric electricity. He requested that the physical cabinet should be moved to another location in the castle, being closer to his living rooms, because the observer should be able to use the instruments between the regular observing hours in case special meteorological events were occurring:
Would Your Serenity be pleased to install the meteorological instruments just mentioned in the physical cabinet, in order to start observations during the new year at this place in connection with other designated locations of your states; the next summer would be most suitable, because the preparation needs time and my lectures [public academic lectures] will start in autumn.
(undated letter 1779 in Generallandesarchiv Karlsruhe, Sign. 77/6400).
At this moment, Hemmer obviously thought only about setting up a national network intended to be similar to that in the neighbouring state of Baden. Stephan von Stengel now became the spiritual father for extending the network to an international dimension. This international network should be supplemented by regional networks in the Palatine and in Bavaria under the guidance of the academies in Mannheim and in Munich. In addition, Hemmer organized in the Palatine a phenological network with 94 observers (Cappel, 1980b), underlining the expectation to learn more on the influence of meteorology on agriculture.
Toaldo had emphasized the importance of atmospheric electricity for plant growth and that people would have noticed that electricity starts to form with sunrise and ceases with sunset: “We can conclude that scattering of the sunrays stimulates the formation of electricity, which moves to the earth taking vapor with it”. Toaldo knew of studies on atmospheric electricity performed in France; however, he relied upon unproven effects. In order to achieve progress, Hemmer proposed that electricity should be measured at meteorological stations, and he constructed an atmospheric electrometer for regular observations using the available experience. The first instrument was designed in 1749 by the clockmaker Jean-Baptiste Le Roy in Paris. Hemmer partly constructed his instrument according to the electrometer of Nollet (1753, Plate 4). Some people claimed to know that atmospheric electricity would be necessary for activating and vivifying the semen of plants (Stoll, 1790). Poey (1879) had compiled a number of publications from the 18th century on electrical effects on germination and growth of plants, including those by the famous Linnaeus. This shows that Toaldo was not alone with his opinion, and Hemmer might have had knowledge of some more literature which would have confirmed his intention to measure atmospheric electricity.
The reason for the wide reception of Toaldo's ideas was the apparent evidence of astrometeorological effects of the moon on weather. Ultimately, this was a consequence of Newton's mechanics, seeing the world as running like clockwork. Toaldo claimed to have shown that atmospheric pressure was higher when the moon had its minimal distance from earth and vice versa. Due to the 19-year saros cycle, according to which the moon takes the same position, weather was expected to become predictable to some degree. From today's statistical point of view, this finding proved to be completely insignificant; however, statistical methods did not exist at the time. In particular, Toaldo had not defined criteria on what should be understood by saying “the weather has changed or not changed” at full moon (Sheynin, 1984). However, everybody was impressed and believed in the effect of the moon on the air pressure, as was well known from the oceanic tides. Toaldo's influence was so large that Hemmer mentioned him in the foreword of the first volume of the Ephemerides.
Today we know that a similar large effect in the atmosphere would require the incompressibility of the air as a prerequisite (as with water), which, however, is not the case. Nevertheless, von Stengel and Hemmer expected to be able to predict weather by analysing periodical fluctuations in time series if they were only long enough. Stephan von Stengel, being a member of the Bavarian Academy of Sciences, initiated a prized question on the effect of the moon on atmospheric pressure and they corresponded with Eberhard Schroeter in St Petersburg (then Petersburg), who had developed a sophisticated method of calculating the aspects of planets and their influence on weather (Bavarian State Library, codex Cgm 6101; Winkler, 2020, p. 44).
3.1 Instrumentation and instructions to observers
Hemmer, being aware of the deficiencies in Böckmann's strategy, chose a more successful way (Hemmer, 1779): he ordered the glassblower Carlo Artaria, who lived in Strasbourg and who had needed an overly long time in manufacturing the instruments for Baden, to come to Mannheim and produce the instruments for the Societas Meteorologica Palatina under his own survey. Hemmer knew that harmonizing instruments needed special care, which the usually freely working glassblower did not consider: the tube of the thermometer must have a uniform calibre; the barometer must be completely free from air bubbles which easily tended to adhere at the inner surface of the glass tube when quicksilver was filled in and which he carefully removed by means of a thin iron wire. By this precaution, he wanted to ensure a perfect vacuum. Hemmer preferred the Réaumur temperature scale to that of du Crest (Ducrest), Fahrenheit, de Lisle (Delisle), and others because the calibration could be reproduced most suitably. He knew that the boiling point of water depends on atmospheric pressure and took care to set the relevant mark at the thermometer to average pressure at sea level.
A barometer with reduction thermometer, two thermometers for use in the shadow and “in the sun”, and a goose-quill hygrometer belonged to the instruments distributed by the Societas Meteorologica Palatina, which were provided at the expense of the Elector Karl Theodor. Declinometers, fabricated by Brander in Augsburg, were distributed to those who were interested. They should be placed on a table of marble, being free from vibrations. A description of all instruments as well as how carefully they were manufactured and calibrated was published in Hemmer (1782a).
In his “Monitum”, i.e. the instruction for the observers, Hemmer recommended the use of additional instruments like precipitation and snow gauges, an atmospheric electrometer, evaporimeter, and a wind vane, but he left it to the engagement of each observer. A universal time did not exist. Observation should be made at 07:00, 14:00, and 21:00 LT, local mean solar time; however, not all stations followed this recommendation (e.g. in St Petersburg the barometer was read at 06:00, 12:00, and 18:00 LT and the thermometer at 06:00, 14:00, and 22:00 LT). The amount of precipitation should be measured at 14:00 LT. A good clock should be available, and the observers must be able to adjust it to the local mean solar time using a meridian line and a correction table. The universal time was introduced in 1884 (in Germany in 1893). Uniform symbols were used to notify special meteorological events. At this time, many observers created their own symbols (e.g. Jacob Nicolaj Wilse in Norway; Federhofer, 2001). Hemmer's meteorological symbols continued to be used in the US army after 1816 (Chenoweth, 1996). In case of being unable to make an observation, another trained person should read the instruments. Additional observations should be noted at the beginning and end of extreme situations. The state of the heaven had to be observed as well as meteorological events like rain, snow, fog, hail, halos, cloud cover, polar lights, the levels of nearby rivers, and the phase of the moon. Botanical and respectively phenological and ornithological observations should be made as well as statistical information on the number of births, deaths, and illnesses of the local population; Hemmer's letter is transcribed in Cappel (1980a). The hope was to recognize meteorological conditions that would favour illness. The Latin language should be used. All stations should provide a short description of the location and the position of the instruments as well as their height above ground. Essays and papers on special findings were welcome. Every half year, the monthly tables should be sent to the permanent secretary.
According to Hemmer's recommendation to measure additional parameters, the observer of Hohenpeissenberg informed the community of his newly constructed evaporimeter or “atmidometrum” (Schwaiger, 1783).
Electricity was the most important research field for physicists in the second half of the 18th century. Atmospheric electrometers were operated at several places (Mannheim, Hohenpeissenberg as mentioned, and a Cavallo-type electrometer was operated by Adriaen van de Perre in Middelburg because in the Netherlands effects of natural electricity on the healthy and ill body were of interest; Zuidervaart, 2006). The observation at an electrometer was dangerous, as the death of Richman in 1753 had demonstrated, who was hit by a lightning stroke when observing his instrument. Despite this negative experience, the observations were impressive. Hemmer's electrometers at Mannheim and Hohenpeissenberg allowed him to measure the spark length at a gap of the antenna and thus conclude on the electrical field strength. Hemmer also had a method to distinguish whether a lightning stroke went from cloud to earth or from earth to cloud. The Hohenpeissenberg station at around 1000 m height gave insight into atmospheric electricity aloft, and the noise of strong sparks at the electrometer gap could be heard throughout the large building, having three floors.
At this time, it was unclear whether electricity would be necessary for the formation of hailstones (e.g. Seiferheld, 1790). A simple phenomenological hypothesis was based on the argument that hail would occur only in electrified clouds, i.e. in which lightning was observed. Bertholon (Academy of Montpellier), a well-known expert on electrical phenomena, reported experiments of Nollet, who had covered the bulb of a thermometer with a piece of linen saturated with spirit of wine. Then the thermometer was electrified by means of an electricity-generating machine, and he could “prove” that this thermometer cooled much more than an un-electrified thermometer. Another conclusion was that lightning rods would drain the electrical fluid from the atmosphere and consequently would help to prevent hail formation. He also argued that lightning rods would rebuff thunderstorm clouds (Bertholon, 1792). Nollet claimed contrarily that lightning rods would attract flashes, which was denied by Hemmer. These few examples illustrate the need for continuous observations on atmospheric electricity. However, science was still in a state where electrical and magnetic phenomena were observed but by far not interpreted correctly. Many basics had to be studied and discovered before progress could be achieved. Priestley had cited Beccaria's opinion that strong electricity would bring hail pieces closer together than snow crystals (Priestley, 1767). Hail clouds therefore would appear much darker than snow clouds or rain clouds. On the other hand, he described an experiment performed in 1767 where he could not accelerate the freezing of water by electrifying it. These few examples show that many conclusions were amateurish and circumstantial, but scholars felt convinced since the majority believed in them. Progress occurred finally when experiments were repeated by others and logical doubts arose.
The Palatine instruments were used at some of the observation sites for a long time: at Hohenpeissenberg until 1840, at Prague until 1829 (von Fritsch, 1850; Lamont, 1851), in Cambridge until 1802, and probably also for similar long times at other sites. Samuel Williams in Harvard considered Hemmer's barometer to be correct, and he reduced his old one to the new barometer (Middleton, 1964).
Some of the instrumental deficiencies were known to Hemmer; others were unknown, such as the rising zero point of the thermometers due to shrinking of the thermometer bulb which, was first realized and described by Bellani (1808) and von Yelin (1824). To overcome the incompleteness of the goose-quill hygrometer (Retz, 1786), Hemmer launched a prize question by the Mannheim Academy of Sciences in 1783. The prize was awarded to Giuseppe Toaldo and his nephew Vincenzo Chiminello in Padua. The main unsolved problem was the availability of fixed points. Chiminello had developed a procedure to take into account the thermal expansion of mercury (for details, see Cocheo and Camuffo, 2000; Camuffo et al., 2014). However, the goose quills were not perfectly round, and their volume individually changed with temperature such that each instrument would have required an individual correction. In addition, we now know that the adaptation time upon a rapid change of humidity is a function of temperature (Winkler, 2015). Hemmer also gave advice on how to construct wind vanes in such a manner that they easily follow the wind direction.
The available instruments were not sufficient in order to describe all processes or effects of meteorology in nature or on human health. Therefore, observers should note agricultural phases like blooming of fruit trees; growth and harvest of crops; and especially nutrient plants, bird migration, illness of cattle, level of rivers, floods, and extraordinary events. In addition, the number of births and deaths of the local population and various diseases should be reported.
3.2 Organizing and establishing the network
Hemmer and von Stengel knew about existing weather observations at many places (Marseille 1749; Berlin 1721, St Petersburg, Göttingen, Padua, and others). They selected those which seemed to be reliable and were operated continuously. The central station (Fig. 4) was established in the Castle of Mannheim with Hemmer as observer and secretary. He wrote invitation letters to more than 50 potential institutions, and he was assisted by diplomatic connections. To implement new stations, invitation letters were not sufficient, but the observers had to be approved, and paid by the superiors and diplomatic support was necessary.
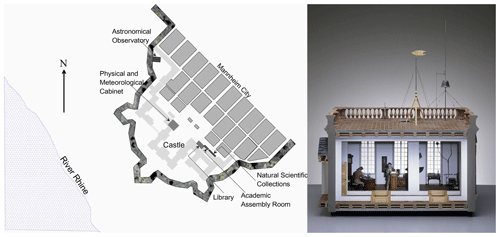
Figure 4Central station of the Societas Meteorologica Palatina in the Castle of Mannheim (historical situation showing the city wall, the castle, and parts of the adjacent city). A model of the meteorological cabinet is available in Technoseum, Mannheim (photo: Klaus Luginsland). The antenna of the atmospheric electrometer is seen on the roof to the right of the wind vane.
Von Stengel travelled through Bavaria and won six monasteries for cooperation (von Stengel and Ebersold, 1993; von Martius, 1859). These stations delivered data starting from 1781. Due to numerous diplomatic and scientific connections, he and Hemmer selected international stations, but they also refused the participation of interested scientists when the distance was not large enough (Cassidy, 1985). In 1785, Hemmer mentioned, in an overview of the first years of activities, that he had obtained support by many influential men in other states and countries (Hemmer, 1785/1786).
-
In Rome the papal librarian cardinal Zelada and the electoral ambassador Count Antici helped to install the observer Calandrelli. Both were members of the Academy of Sciences in Mannheim.
-
In Bologna, the Count of Savioli, a member of the Academy of Sciences in Mannheim, arranged the meteorological station in Bologna with Matteucci as observer.
-
The Capuchin general Erhard von Radkersberg, living in Munich since 1779, agreed that monks of Milan should do the observations at the hospice on St Gotthard in Switzerland.
-
Baron von Dalberg in Mainz, also governor of Erfurt, agreed that meteorological observations were to be performed at the University of Erfurt.
-
The Baron of Beveren arranged the station in Düsseldorf.
-
Mr David, electoral agent and secretary of the legation in Paris, supported the installation of French stations.
-
Bugge in Copenhagen supplied the station in Spydeberg with instruments rebuilt after Hemmer's models, and the station in Moscow obtained rebuilt instruments from the Academy of Sciences in St Petersburg.
Van de Perre in Middelburg bought instruments from Hemmer at his own cost, because, as a private man, he could not guarantee uninterrupted observations. This shows how careful Hemmer and von Stengel selected stations for the European network. Thus, the society adopted a coordinating and standardizing function in unifying the scattered observations and providing instruments from the same manufacturer. This new and outstanding function was even emphasized and recognized by the philosopher Schelling (Schelling, 1861, p. 492).
The monastery Polling in Bavaria was also interested in participating in the network but was rejected. In his reply, Hemmer stated that the meteorological society is not allowed to sell any instruments against payment. He would only have helped the Duke of Saxony-Gotha and van de Perre in Middelburg with paid instruments (Hemmer, 1782b).
All observers were nominated as external members of the meteorological class of the Academy of Sciences in Mannheim and listed regularly in the “Kurpfalzbaierischer Hof- und Staatskalender”.
The very successful organization and detailed description by Hemmer, e.g. how the instruments were manufactured and calibrated, stimulated other academies to imitate the organization of national meteorological networks: in 1785, the Mannheimer Zeitung (15 November) reported that the Swedish king had concluded to establish a meteorological network in his kingdom after the model of the Societas Meteorologica Palatina (Mannheimer Zeitung, CXXXVIII, 15 November 1785). The Swedish Academy of Sciences had made a corresponding proposal.
Similarly, the Academy of Sciences in St Petersburg achieved that the Russian emperor agreed to establish a meteorological network in all Russian provinces and to provide instruments built after the models of Hemmer (Hemmer, 1785/1786). In Spain, there was hope to include Madrid as a new station, and instruments had been sent to the minister of state Florida Blanca (Hemmer, 1785/1786). However, this aim was not realized for unknown reasons. Also, in India, initial ambitions to arrange meteorological stations could not be realized.
Some of the invited persons refused after having firstly agreed to do observations: Georg Christoph Lichtenberg (1742–1799) in Göttingen, for example, primarily confirmed his participation and was very interested to obtain a hygrometer, which he was lacking and which he needed for his lectures (Lichtenberg, 1985, Vol. II, undated letter no. 760, [1780]).4 Later he urged his colleague Gatterer, who was already performing observations, to take over this duty. He also promised to ask his friend Jean Deluc in London, although being unable, in his position as lecturer of the Queen, to observe personally, for helping to find observers in England. No success could be achieved with British stations, although the Academy of Sciences in Mannheim had nominated scientists in Dublin and Edinburgh as members (Cassidy, 1985). Scholars from Lemberg, Lisbon, London, Paris, Turin, and Vienna were also invited, but they did not participate.
Gatterer from Göttingen, in his turn, rated Wilse in Norway to be unable in systemizing the complex processes of the atmosphere.5
The instruments like barometer and thermometer were sent out in duplicate, because many of them broke during shipping. Printed tables were distributed to facilitate and harmonize the notification. The Mannheim hours were not obeyed at all stations, as already mentioned. The printed tables should also help to achieve a high uniformity and completeness. The notification of positive and negative temperatures was explained. A special piece of advice was given to avoid errors in thermometers by the radiation of candles which were needed in winter or at night to read the outside thermometers.
Although the instruction was detailed, some of the stations kept the window of their observing room open during fine weather.
The observations were delivered to Mannheim not by the ordinary post but through diplomatic channels.6 Any risk with regard to a possible loss of data should be avoided.
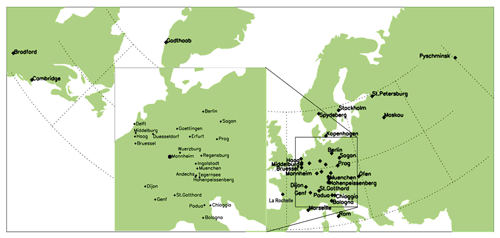
Figure 5Map with stations of the network of the Societas Meteorologica Palatina (1781–1792). Table A1 in the Appendix lists the stations and the duration of observations.
A total of 57 stations were invited to participate in the network, and about 20 of them sent in observations in the first year (Voss, 1994). Data were published. Finally, data of only 39 stations were printed, some of which reported only for a few years (Fig. 5; Table A1 in the Appendix). The reasons why another 18 stations did not send in data were complex: at some of them, only broken instruments arrived, whereas others like London or Vienna might have had no confidence in the success of the project. In the first volume of the Ephemerides, the letters of confirmation were published. Père Louis Cotte in Paris, although having agreed to contribute to the network, never sent in data, probably because of broken instruments.7
Von Stengel succeeded in convincing the prelate of the Bavarian monastery of Rottenbuch, being responsible for the operation of the station Hohenpeissenberg, to install another atmospheric electrometer (Bertucci, 2007; Winkler, 2005). He personally brought the fragile barometers and thermometers to some of the Bavarian stations.
At some of the places, meteorological observations already existed, although with interruptions, e.g. in Berlin (1719; Cubasch and Kadow, 2011), Bologna (Camuffo, 2000), Göttingen (Gatterer, 1780), Copenhagen (1767), Geneva (1753; Häderli et al., 2020), Marseille (1749), Regensburg (1773), Padua (1725), St Petersburg (1751), Prague (1775), Stockholm (1756; Moberg et al., 2002), Sagan (1773; von Felbiger, 1773), and Würzburg (1773; von Rudloff, 1967; Camuffo, 2000; Winkler, 2020). The new society thus coordinated these activities.
Phenological observations were initiated by the Societas Meteorologica Palatina in many countries like Belgium (Ihne, 1894; Demarée and Chuine, 2006), Italy, France, Norway, and Russia or supplemented in Germany, Switzerland, Sweden, and Finland (Cappel, 1980a). In Mannheim, the military major Denis started phenological observations in 1783.
At some stations, physicians studied the disease pattern of the local population: Melchior Güthe and Johann Nepomuk Fischer in Mannheim, Winkler in Regensburg, Cloos in Stockholm, and van Breda in Delft (Hemmer, 1785/1786). They provided the requested data.
The network became well known and stimulated scientists to report data from additional stations outside the meteorological society, e.g. meteorological data from the African isles of France (Mauritius) and Bourbon (Réunion) were reported by Martin Brunel (Brunel, 1790), member of the Académie des Sciences de Bréziers, and data of Puna (previously known as Poona) in India were reported by the Belgian scholar Malavois (Ephemerides 1788; Malavois, 1790, p. 400). In 1791, Brunel was nominated as an external member of the Academy of Sciences in Mannheim (Voss, 1994).
Both the regular instrumental observation and the observation of the state of the heaven turned out to be most important for scientific progress. Although not published in the Ephemerides and although many interpretations were later found to be wrong, meteorological phenomena were discovered and described, e.g. the thunderstorm hook (Heinrich, 1794). Another point was the overcoming of religious practices in handling natural hazards, e.g. ringing church bells or shooting guns in order to disperse thunderclouds.
Because Hemmer's organization and management of this station network had previously turned out to be exceedingly efficient based on steering the individual stations from a central station, which also collected and published the data, it became a model for later meteorological networks and other networks, e.g. Alexander von Humboldt's Magnetic Club (1829–1834) and that of the Magnetic Association of Göttingen organized by von Humboldt and Carl Friedrich Gauss in 1834 in which comparable instruments were observed at given times (Reich, 2023).
3.3 Expenses to print the Ephemerides
The costs for paper and printing of the first three data volumes were very high and appreciably stressed the academic fund for other tasks (Archive of Bavarian Academy of Sciences, Sign. VII, I 26, l: fol. 4.):
Descriptio Instrumentorum | 28 Gulden (florines) 32 Kreuzer |
Ephemerides 1783, Vol. I | 1285 Gulden 22 Kreuzer |
Ephemerides 1784, Vol. II | 1604 Gulden 21 Kreuzer |
Ephemerides 1785, Vol. III | 1942 Gulden 35 Kreuzer |
To rate these expenses, we can compare them with Hemmer's yearly salary as secretary of the meteorological society, which amounted to 300 Gulden. The whole budget of the academy amounted to 6000 Gulden, from which 1200 Gulden had to be paid to other societies. The budget was not raised when the meteorological class was arranged. Interests arising from the foundation capital could not compensate the expenses of the meteorological class. After summing up the salaries, only 1500 Gulden remained for printing the meteorological ephemerides. Thus, it became difficult to publish the data of all stations in extenso in each year. Clearly, a single academy was financially overwhelmed with the high printing costs of this European project, but no effort was undertaken for sharing the costs among more participants.
To remain able to print the following volumes, the number of stations from which the data were published was either reduced or the data were not printed completely but only with daily or monthly means. When in later years the financial resources reduced further, the members of the academy abdicated their weekly fee for attending the sessions (Fuchs, 1963).
Progress was slow and small; scientific meetings could have been helpful but were too difficult to organize. Instead, scientific academies in numerous cities were allowed to discuss findings and scientific questions. The local view on the weather still prevailed and large-scale (synoptic) pressure systems and their movement were unknown.
The great interest in observing nature stimulated engagement and participation, although most scholars of that time can be compared to unexperienced pupils and adolescents who are in the process of learning and who make many mistakes and misinterpretations, because the magnitude of effects are overinterpreted.
Lalande (1803) mentioned in his Bibliographie astronomique the 10th volume of the Ephemerides for the year 1790, printed in 1793. He regretted very much the reduced size of the volume comprising only 319 pages, whereas the volume of 1783 had 800 pages. For scholars, the completeness of the observed datasets was much more important than the commercial aspects.
3.4 Hemmer's death and the demise of the Societas Meteorologica Palatina
On 28 April 1790, Hemmer suddenly suffered from a weakness attack (diagnosed as “hypochondriasis”) while he was installing a lightning rod. He died a few days later on 3 May at the age of 56 years (Budde, 2014). An autopsy showed heart problems, causing a stroke (Anonymous, 1915). During the past 2 years he had suffered from palpitations. The engine of the meteorological society was now without steam. Ten of Hemmer's scientific papers were printed in the last physical volume of the Acta Academiae Theodoro-Palatinae in 1790.
After Hemmer's death in 1790, no new secretary of the meteorological society was nominated. As editor of the Ephemerides, the physician Melchior Güthe (1753–1812) was employed. The engagement and energy, which Hemmer had brought in, was missing, and the whole project slowed down. Güthe even did not take care of the observations in the meteorological cabinet and had no interest in the physical cabinet, and he refused to take over the correspondence (Stephan, 2017). Stephan von Stengel deplored this development very much (von Stengel and Ebersold, 1993), but neither he nor his father was in a position to bring the Academy of Sciences in Mannheim back to the earlier efforts in meteorological research. A successor with the personal engagement of Hemmer could not be found.
Soon after Hemmer's death, the situation of the Academy of Sciences in Mannheim deteriorated more and more. The budget was based to a great part on interest income from mortgages being invested in several hypothecary credits created mainly on the left side of the Rhine. When, in the beginning Napoleonic Wars, the French troops advanced in 1792 to the Rhine the academic budget diminished dramatically (Traumüller, 1885; Kreutz, 2014): already since 1791 no prize question could be issued. Since 1793, vacant positions at the academy were not filled again, and since 1794, when the left side of the Rhine was occupied completely, no volume of the academic series Acta Academiae Theodoro-Palatinae could be printed. Thus, personal and financial bottlenecks induced the demise of the meteorological society. In the meantime, it had become evident that periodic variations in atmospheric pressure by the moon were very small, and the hope of being able to make a meteorological prognosis had to be abandoned. The Academy of Sciences in Munich, operating a regional network, ended this project in 1800 with the argument that the observers had only provided a collection of data (Winkler, 2020; protocol of 4 February 1800). Maybe, after Karl Theodor's death in 1799, anti-academic forces in the new government in Munich had requested to terminate the project.
In November of the year 1795, the western wing of the castle of Mannheim was set on fire by a shot from Austrian troops. In this part, the meteorological cabinet was destroyed, including the archive with the irreplaceable original meteorological tables – a terrible loss for science. This violent destruction of the centre of the meteorological society implied the end of the coordination and collection of the tables (Budde, 2014).
It is astonishing that in 1802, seven years after the last volume of the Ephemerides was printed, the “weather society” with all national and international members was still mentioned in the official calendar “Kurfürstlich Pfalzbaierischer Hof- und Staatskalender auf das Jahr 1782” (1802), which means that the Societas Meteorologica Palatina formally still existed, although being inactive for many years and although the founder, Karl Theodor, had died in February of 1799.
4.1 Data quality
Since the measurement of atmospheric pressure was regarded as more important than that of ambient temperature, some of the stations, like Regensburg (Ratisbona) and Hohenpeissenberg, kept the window of the observing room open during fine weather for the first years as mentioned above. Thus, temperatures measured in a screen at an open window could be partly biased by air from the interior of the building.
The data collected in the 12 Ephemerides volumes have often been considered representative for the pre-industrial period, especially with regard to obtaining information about climate change. However, this somewhat indiscriminate adoption of these data needs to be scrutinized; with respect to the temperature records, it has been overlooked that the zero point of thermometers was not stable as mentioned above. Hemmer, although he did not know of this effect, emphasized from experience that it was necessary to manufacture all instruments from the same glass. In the 1820s, this effect was discussed broadly, and most physicists attributed it to the atmospheric pressure which would compress the evacuated thermometers (e.g. von Yelin, 1824). In the 1880s, the instability of the glass could be attributed to the glass mixture. Mixtures for thermometers were optimized, and the cause for the secular rise of thermometer zero points was better understood (Krause, 1974; Winkler, 2009a, b). This secular rise of the zero point was of the order of 0.65 ∘C in the first 5–6 years (Lamont, 1851; Winkler, 2009a). Nevertheless, many homogenizations were performed without considering the instrumental bias. In 1826, the zero point of the thermometer in Stockholm was checked and found to be too high by 0.75 ∘C, unfortunately without notifying the period in which this thermometer was used (Moberg et al., 2002). The recognition of the instable zero point of thermometers did not lead to corrections of past data at all stations. Recently, Pappert et al. (2021) have applied these findings for correcting and homogenizing temperature and pressure observations of the Palatina network to make the data more usable for climate change issues.
Other instrumental deficiencies and uncertainties of historical thermometry are described, for example, in Pelz (1993). Brander, as an experienced and thorough manufacturer of thermometers had already arranged an intercomparison with other manufacturers (Christin in Lyon, France, and König in Franecker, the Netherlands) in 1757 and achieved good agreement (Winkler, 2017). But even when temperatures of neighbouring stations agree well, the thermometers might suffer from a similar rise of the zero point.
Other instrumental deficiencies were recognized earlier: barometer deterioration due to oxidation of mercury or by penetration of air into the vacuum. The capillary depression was studied by Pilgram (1788), but no conclusions were drawn on the barometer reading technique or on necessary corrections to be applied. It was also unclear whether the mercury position should be read at the top of the meniscus or at the edge of the mercury column at the glass wall. Later it turned out that the height of the cap may vary with time, depending on the chemistry of the glass influencing the contact angle, because the glass changed its volume due to slow diffusion effects in the crystalline structure of sodium and potassium ions. This is important when homogenization is undertaken.
The hygrometers in use provided some coarse information on humidity. For the goose-quill hygrometers, Chiminello in Padua had developed a method for compensating the thermal expansion of mercury on its volume (Cocheo and Camuffo, 2000; Camuffo, 2000), and he won half of the corresponding prize of the Academy of Sciences in Mannheim in 1783 (Hemmer, 1783/1784). However, a uniform volume change of goose quills with temperature can be expected only when they are more or less perfect in roundness (Winkler, 2009b). Placidus Heinrich in Regensburg performed comparisons of goose-quill and gut-string hygrometers (Fig. 6), which showed that the goose-quill hygrometer reacted relatively linearly, whereas the gut-string hygrometer did not.
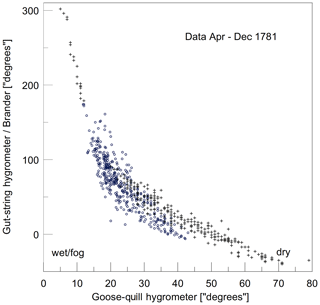
Figure 6Comparison of goose-quill vs. gut-string hygrometers in Regensburg, 1781 (crosses: April–May 1781; circles: September–December 1781). Although Hemmer knew the gut-string hygrometer, he preferred the goose-quill instrument, which had a better linearity with humidity (Winkler, 2020).
The magnetic needle in the declinometer could be disturbed by iron pieces near the instrument. Such a disturbance was unknown at that time.
Despite these instrumental deficiencies, a multitude of valuable information on basic meteorology, weather, and climate has been derived from the data pool collected by the Societas Meteorologica Palatina.
4.2 The Ephemerides and scientific results presented therein
In the Ephemerides, the data were published partly in extenso and partly as monthly means. For a long time, this collection of data was one the most reliable basis for scientific use (Fig. 7).
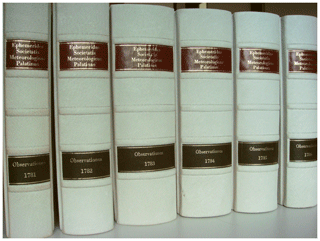
Figure 7Ephemerides Societatis Meteorologicae Palatinae (photo: Peter Winkler, Observatory Hohenpeissenberg, 2006).
Short reports on special themes were also printed in the Ephemerides as shown below. In most cases, only observations and their interpretations were reported. Real physical understanding was low at that time; however, physical methods were applied.
-
Ephemerides 1783 (Vol. IV; Hemmer, 1785): Hemmer wrote on the dry fog over Europe in 1783, not accepting the widespread hypothesis of its volcanic origin. The availability of a hygrometer allowed him to conclude that the turbidity of the air was not caused by water droplets (Stephan, 2017; Cappel, 1980c).
-
About the same item, more opinions were presented by Koenig (1785), Maret (1785), Senebier (1785), and van Swinden (1785). Holm (1785) described the eruption of Laki in Iceland and its destructive effects.
-
Other scientists argued that the dry fog originated from the earthquake in Calabria in February 1783 (Torcia, 1784). At this time, no information and even no imagination existed on the strength and direction of the upper airflow. As a consequence, erroneous conclusions were drawn easily.
-
Planer: diurnal pressure variations based on readings every 4 h were attributed to the effects by the moon, atmospheric electricity, and wind.
-
In the second volume, Cölestin Steiglehner published a comparison of the barometric pressure readings at three stations (Regensburg, London, and St Petersburg) between December 1775 and January 1776. Based on these data, he recognized the eastward propagation of pressure minima. Steiglehner's primary idea was a study of the number and mean duration of “pressure oscillations” per year which he adopted and continued as a similar approach of van Swinden in the Netherlands. He hoped to be able to predict the pressure tendency for the next 3 h as argued in another private publication (Steiglehner, 1783).
-
Samuel Williams presented yearly barometric and thermometric maxima and minima from Bradford (USA) for the years 1771 to 1776. Wigglesworth presented monthly barometric and thermometric maxima and minima of the years 1781–1783.
-
In the Ephemerides 1787 (Vol. VI), van de Perre published observations of tides at Middelburg, Vlissingen, and Westkapelle (formerly Westcapella).
-
Hemmer presented hourly data of atmospheric pressure monitored with the recording barometer of Changeux in Ephemerides Vol. VI, containing the data of 1788, and he became aware that a daily cycle existed which, therefore, he could only attribute to influences by the sun but not the moon (Hemmer, 1790).
-
Chiminello had calculated daily courses of the temperature of Padua for the years 1788 and 1789 (Ephemerides 1789, printed 1793), and Toaldo presented average temperatures of several cities in Italy, including Rome, Chioggia, and Padua; however, only the number of years of observation were given but not the overall period.
The attractiveness of the Ephemerides was obviously large, and many additional observations could have been accepted for inclusion. However, the printing costs were very high, and the volumes sold less frequently than expected.
After Hemmer's death in 1790, the physician Johann Melchior Güthe (1753–1811) edited the following volumes, without investing the necessary energy or enthusiasm. Thus, Vols. XI and XII appeared only in 1794 and 1795, respectively.
The Bavarian and many international stations continued their observations until 1803, when the monasteries were secularized, except Hohenpeissenberg, where the observer was obliged by the ministry to continue the observations without interruption. Many other stations of the network continued the observation programme as well.
In 1806, the Academy of Sciences in Mannheim was closed and the remnant documents transferred to Munich. Among these were the lead letters with the symbols for typesetting (Archive of the Bavarian Academy of Sciences, Sign. VII 453–454).
4.3 Early and modern scientific evaluations of the meteorological observations
The collected data found a broad interest very early: Thomas Jefferson, the later president of the United States, while travelling through Marseille in 1787, asked his correspondent William Short in Paris in a letter of 5 May to buy for him the first volumes of the Ephemerides (Jefferson, 1983). He might have been informed about them during a visit to the academy or the astronomical observatory of Marseille. The data of the meteorological society were evaluated very often, which shows the exceptional importance of the Ephemerides. The data have been used in numerous textbooks, especially for presenting climatological information (Kämtz, 1831, 1832, 1836). Only a few examples of the scientific outcomes are listed below, without claim of completeness, and shall illustrate this.
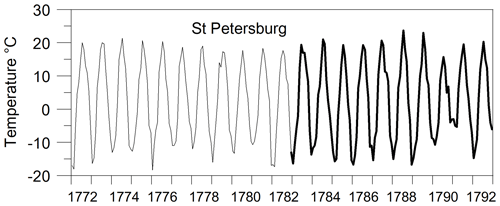
Figure 8Monthly mean temperatures of St Petersburg. The bold line shows the data taken from the Ephemerides of the Societas Meteorologica Palatina; the data of the period 1772–1782 (thin line) were taken from Novi commentarii Academiae Scientiarum Imperialis Petropolitanae (Heinrich, 1813).
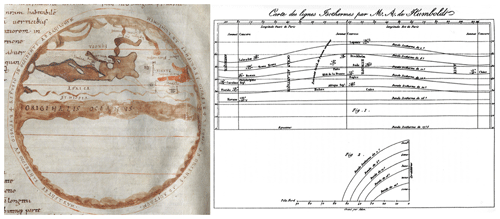
Figure 9Left: example of a traditional Macrobius map (Bavarian State Library, Clm 6362; fol. 74r, miscellany), containing Macrobius' commentaries on Cicero's “The Dream of Scipio” (11th century; a similar map in Clm 15378, fol. 217r, 15th century); it also shows five climate zones running parallel to the Equator. Right: von Humboldt's isotherms in 5 ∘Ré distance (von Humboldt, 1817) in the section 90∘ W to 120∘ E; the central vertical line marks 8∘ E. Continents are not indicated. His analysis showed that the isotherms do not run parallel to the Equator. The lower panel shows the dependence of temperature on height (a.s.l.) and, correspondingly, on geographical latitude.
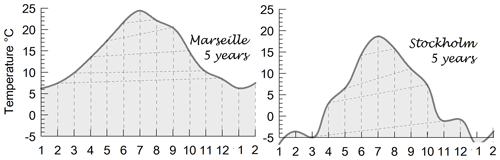
Figure 10Average yearly cycles of temperature at Marseille and Stockholm according to Schön (1818). The y scales, missing in the original, have been supplemented and harmonized, and the units have been changed to ∘C. Schön connected months with the same solar elevation by inclined lines, in order to visualize the asymmetry of the yearly course of the temperature in relation to the sun's elevation.
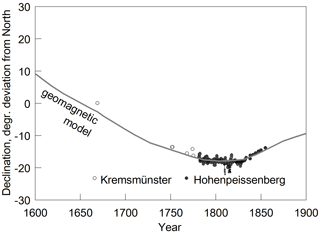
Figure 11Secular variation of the magnetic declination (model and observations) adapted from Korte et al. (2009). Crosses mark unreliable measurements, because the declinometer deviated from the northern direction.
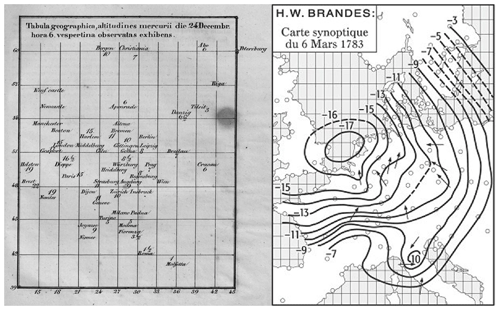
Figure 12Left: first synoptic map of 24 December 1783 based on data from the Ephemerides and supplemented by further observing stations, designed by Brandes (1826, Annex with a total of three maps); numbers show the negative pressure deviation from the stations average in Parisian lines (1 line = 2256 mm Hg); the meridians are given in degrees east of the isle of Ferro, now El Hierro. Right: a reconstruction of the isobars by Hildebrandsson and Teisserance de Bort (1898), using data from the Ephemerides and the method of Brandes for 6 March 1783. In addition, they added reported wind vectors from which the convergence of the air flow to the pressure minima became obvious. Brandes had not yet recognized this physical law.
-
The frequent cold weather in upper Bavaria was wrongly attributed entirely to the proximity of the Alps. However, by an evaluation of temperatures as a function of the elevation of stations, Stephan von Stengel showed that the elevation rather than the distance from the Alps is responsible for the local average temperature (von Stengel, 1786).
-
To facilitate the pressure readings to the prescribed temperature of 10 ∘Ré (degrees Réaumur), Guarin Schlögl (1752–1788), observer at Hohenpeissenberg, calculated tables from which the correction value could be retrieved (Schlögl, 1787). The pressure range, for which his tables could be applied, included the highest station, St Gotthard in the Alps. Although the adopted coefficient of expansion of mercury was slightly too large, these tables helped to avoid errors, when the pressure reduction would be calculated manually.
-
In 1791, the observer Albin Schwaiger compiled a climatology of the station Hohenpeissenberg using the first 10 years (Schwaiger, 1791). This work was stimulated by his abbot, who saw a need for presenting the observations to the public. In 1811, a second but unchanged edition appeared.
-
Père Cotte in Paris published a similar evaluation in 1792 for a number of French stations: barometric variations, numbers of frost days, magnetic declination, and other variables (Cotte, 1792).
-
Gelas Karner (1763–1816), another observer at Hohenpeissenberg, calculated the elevations a.s.l. of eight Palatina stations from barometric data in a very time-consuming work and published the results in 1812 (Karner, 1812).
-
Similarly, Placidus Heinrich from the monastery St Emmeram in Regensburg calculated the height of stations along the Danube down to Budapest (Heinrich, 1812). His results agree remarkably well with modern heights. This was the first paper from a station on the slope of a European river. This work was stimulated by Alexander von Humboldt, whom Heinrich had met in Paris.
-
In 1813 Placidus Heinrich published a climatological study on the temperature of St Petersburg (Fig. 8), for which he had evaluated the data from 1772 to 1792, i.e. including the period covered by the Societas Meteorologica Palatina (Heinrich, 1813). His impetus was to inform scientists at least in Germany about the harsh climate in Russia. In order to enable a comparison with other cities, he shifted the observations to the Gregorian Calendar, being in use in Russia until 1918. He also recalculated the temperature for the scale of Réaumur from the original Delisle's scale. In addition to the monthly mean temperature, he indicated the extremes.
-
An early evaluation of extremes and mean values of heat and cold from the Ephemerides has been conducted by Holyoke, a Massachusetts physician in Salem, USA. He was interested in excess heat and cold of America beyond that of Europe in the same latitude (Holyoke, 1793). His unrealistic conclusion was that the pine forests were causing the cold in America, whereas in Europe the cutting down of forests for many centuries had brought a much milder climate. Being an adherer of the phlogiston theory, he was convinced that huge evergreen pine forests would absorb large amounts of phlogiston, thus permanently producing dephlogisticated, i.e. cold, air. This is a typical example of obstructions in learning processes at that time.
-
An overview of the strong frost in December 1788 has been worked out by Strnad in Prague by evaluating the eight volumes of the Ephemerides. He compared the peculiarities reported from all stations of the Palatina network and calculated cold sums to be able to compare the winter strength in the different regions of Europe (Strnad, 1793). However, no map was constructed.
-
In 1817, Alexander von Humboldt used the temperature data of the Palatine society and some other stations for the first construction of global isotherms (von Humboldt, 1817). It is important to mention that for this construction he applied a reduction of the temperature of elevated locations to sea level and considered data quality as well as the lengths of observation periods. His idea was stimulated by the traditional so-called Macrobius maps of the climatic zones in which the isotherms run parallel to the Equator (von Humboldt, 1837) (Fig. 9). Using observed temperatures mainly from the Ephemerides, Humboldt could show that the isotherms were not parallel to the Equator and that in Europe the isotherms extended further to the north (a consequence of the heat transport by the Gulf Stream, which was still unknown at his time). Von Humboldt's graphical visualization was an important progress as compared to the presentation of data in a simple table.
-
Macrobius was a Latin philosopher of the fifth century who had firstly described climatic zones in his commentary of Cicero's work. Although he hardly can be considered the inventor of climatic maps, this type bears his name. Examples of Macrobius-type maps can be found in many major libraries (Destombes, 1964, 85–95). The validity of the orientation of the climatic zones shown there was not questioned until von Humboldt's analysis.
-
In 1818, Schön in Würzburg published a book on Basics of the Atmosphere and Weather. There, he presented graphs of the yearly variation of meteorological elements (Fig. 10). He also demonstrated, by connecting months with similar solar elevation, the asymmetric deviation of the yearly temperature variation from the sinusoidal solar insulation peaking on 21 June (Schön, 1818).
-
Volney investigated the influence of the sea on the temperature of coastal stations in France in 1821 and recognized the increasing continental influence with the distance from the coast (Volney, 1821).
-
In eastern Europe, the data of the station Budapest were used to extrapolate to meteorological parameters of other stations like Timișoara (Temeswar) (Csernus-Molnár and Kiss, 2011; Csernus-Molnár et al., 2014).
-
Polar lights should be observed in the meteorological network, and Aspaas evaluated the observations, regarding the modern knowledge. They counted about 1400 observations in the Ephemerides, but their verification showed that a large part of them were misinterpretations, because noctilucent clouds were interpreted as polar lights (Aspaas and Hansen, 2012). However, the data were used already earlier to identify the “polar-light-oval” (Fritz, 1874).
-
Since strong solar mass ejection auroras could be seen even in Mediterranean countries, Hattori et al. (2021) investigated simultaneous auroral reports in the Ephemerides 1788 at many stations (Rome, p. 96; Geneva, p. 332; Budapest, p. 354; Padua, p. 363) and compared these records with a large deviation of the magnetic declination at Mannheim. At Hohenpeissenberg, unusual variations of the magnetic needle were also noted. Hattori et al. (2021), who had found simultaneous reports on polar lights in Japan, interpreted them as an extremely strong solar storm disturbing the geomagnetic field before the Dalton minimum (1795 to 1830).
-
The variations of the magnetic needle during polar light events were known before 1750. Hemmer and other observers confirmed this interaction by his own observations (Hemmer, 1792). Wilse in Norway also described such effects (Federhofer, 2014) as well as the observer on Hohenpeissenberg (Bayerische Akademie der Wissenschaften, 1782–1796, Vol. 1, 1783, p. 76). In 1806, von Humboldt termed the effect of polar lights on the magnetic needle as a magnetic storm. The periodicity of sun spots and declination was analysed by Wolf (1861, 1862), using stations of the Societas Meteorologica Palatina.
-
The observations of magnetic declination turned out to be more valuable than originally expected. For example, books on applied geography of Hungary mentioned the historical data (Hunfalvy, 1882). Modern methods allow researchers to calculate the secular change of the geomagnetic field (Fig. 11). The historical measurements were used for verification and quality control (Hunfalvy, 1882; Korte et al., 2009).
-
The pressure observations were used to construct first synoptic maps. The first trial was made by Brandes (1826), who used deviations from the mean instead of the unreduced pressure (Fig. 12). In 1898, Hildebrandsson and Teisserance de Bort (1898), who applied the meantime collected meteorological knowledge, showed isobars in a re-evaluation, and by plotting wind arrows they could demonstrate the convergence of near-surface air into a low. The discovery of this phenomenon is usually ascribed to Dove (1857). More recently, monthly pressure maps for 1781–1788 were calculated by Gisler (1985), and daily pressure maps were drawn by Kington (1988) using the data of the Ephemerides. A similar evaluation considering some improvements was published by McNally (2004). In all cases, the data of the Palatine society provided an important data source.
-
Using the historic observations, the global circulation patterns between 1781 and 1785 have been reconstructed by Yiou et al. (2014), in order to visualize the dispersion of the ash cloud of the Laki eruption in Iceland over Europe in 1783. A thesis at the University of Rennes focused on the same eruption using the Ephemerides (Hellman, 2021).
-
An apparent cluster of floods could be recognized in the mid-1780s. The winters in 1784 and 1789 were extremely cold, and the Palatina network data show these conditions spreading all over Europe: the temperature in Padua stayed below zero for many days in January and dropped as low as −12 ∘C in February 1784. As a consequence, the Lagoon of Venice froze in both years (Camuffo et al., 2017), and Lake Constance in 1789 (Meichle, 1963). The Rhine was also frozen. Due to a rapid warming in February 1784, a centenary flood causing huge damage occurred in many parts of Europe (Anonymous, 1784). In the Ephemerides, such events were not explicitly mentioned, although the observers might have reported them. However, using the published data, the meteorological situation could be reconstructed (Brázdil et al., 2010).
-
A historic storm over the North Sea on 21/22 March 1791, causing a flood in Hamburg, could be re-analysed by Lamb (1991). At this time, the moon had a minimal distance to earth. Historical storm surges in the Adriatic Sea have been re-evaluated by Raicich (2015).
-
The quality of historical precipitation measurements was studied by Braun, who investigated errors due to placing the precipitation collector at different heights above ground. He compared, amongst others, data collected by Böckmann in Baden with those collected by Hemmer (Braun, 1885). A recent attempt to reconstruct a long series of precipitation measurements for the station of Padua was tried by Camuffo et al. (2020).
-
Astrometeorological influences of the moon and the planets on weather as postulated by Hell in Vienna (Hell, 1775) and Toaldo in Padua (Toaldo, 1777b) had stimulated the work of the Palatine meteorological network. This hypothesis was later re-examined by de Lamarck (1798), Cotte (1805), Ellinger (1814), and Celoria (1874); however, due to missing knowledge of statistical significance, they erroneously believed to have proven an effect of the moon on meteorological processes.
-
The temperature measurements of the Palatine network have turned out to be important for comparison and respectively calibration of proxy data derived from tree-ring evaluations (Holopainen, 2005) or from mussel shells (von Grafenstein et al., 1996). The cold year of 1784, already mentioned above, was responsible for an exceptionally narrow tree ring in northern Europe, and also here the data of the Ephemerides were used for confirmation (Kalela-Brundin, 1996). The data of the Palatina network have been used to calculate European maps of the deviation of the summer temperature from the mean (Schweingruber et al., 1991).
-
The synopsis of time series of atmospheric pressure gave insight into parallel developments at various places which paved the way for recognizing the extension of atmospheric pressure systems (Daniell, 1827).
-
Nicander in Stockholm constructed a meteorological table for a 21-year period ending in 1795, i.e. including the Palatina period, in order to investigate the influence of weather on mortality due to different diseases. He was convinced that influenza in Sweden could originate from Siberia and vice versa. When he compared the barometric variations with diseases, no clear results were obtained (Nicander, 1801). This first trial to study meteorological influences on human health was imitated by other scientists. The concept of the Societas Meteorologica Palatina to collect meteorological data together with data of diseases provided the necessary basis. After a comparative study on influenza waves in Europe in the year 1782, about one-third of the population of Rome and nearly all of the population of Regensburg fell sick with this infection (Ripperger, 1892). This information could be based on data of the Ephemerides.
4.4 Poor knowledge and lessons to be learnt
Due to the prevailing focus on the local weather, no information existed on large-scale pressure systems or on wind systems in upper atmospheric levels. Thus, the data were often prone to misinterpretation. As mentioned earlier, the dry fog in the year 1783 originated from the volcano Laki in Iceland. Many scholars had doubted that the ash could be transported over such great distances. Professor Lichtenberg at the University of Göttingen, who thought about the unusual dry fog, was convinced that it was responsible for frequent thunderstorm formation. He termed this mysterious fog as “nebula nebularum” and investigated the air quality but found no conspicuous change of the properties (Hochadel, 2009). Therefore, he supposed that the air might have lost some of its constituents, because they were washed out by rainwater. Being unable to find a convincing explanation, he wished that the strange fog would have stayed in Calabria, where he assumed its origin.
Another misinterpretation was published by Hemmer in 1790: he had proven in an experiment that a flame would produce free electrical charges. This led him to conclude that a fume from a house was strongly electrified and had attracted parts of a cloud passing that place (Hemmer, 1790).
Schön in Würzburg also studied the results of the meteorological society, being stimulated by Heinrich in Regensburg. He found the pressure variations to increase with increasing latitude (Schön, 1818; Daniell, 1827). Because no stations in high latitudes were available, he concluded that the barometric variation would generally increase with increasing latitude.
A very important progress for science during the enlightenment era was the edition of numerous journals in all countries. Here, new ideas were scrutinized, and the interpretation of experiments was questioned. When studying historical papers, we easily recognize that most scholars were well informed about the state of knowledge. The data of the Palatina network were used immediately after appearance with many attempts for interpretation and gaining understanding. For example, Rosenthal (1784/1785) compared the temperatures of the two neighbouring stations (Hohenpeissenberg, ca. 1000 m, and Andechs, ca. 700 m) and found the pressure difference to be not constant in the yearly course. He concluded that the atmospheric pressure data could be doubtful. He did not know that in winter Andechs was frequently lying within the cold valley pool where the pressure was higher due to the greater density of air, whereas Hohenpeissenberg was mostly above the topping inversion. Despite these misinterpretations, the harmonized instruments provided correct results, and the exploration of new unexpected phenomena led to scientific and methodological progress.
Other scholars were glad that, by such an observation programme as set up by the Societas Meteorologica Palatina, antiquated opinions would be overcome, such as: “the atmosphere and especially clouds should be regarded as the footrest of God” (Ernesti, 1773; Hollmann, 1783). Such opinions were deduced from bible translations and still taught by theologists who educated the pupils and students. Thus, the observation of meteorological phenomena was an important step to a physical view on nature.
The available instruments misled some scholars to believe in meteorological effects which in fact did not exist as such. Although many errors and misinterpretations occurred, the database in the Ephemerides remained available and was used by persons who had not been observers in the network. More political insight into the usefulness of such a long-term and diverse data collection is needed with respect to its fostering of both progress in science and education for the general benefit of society. However, decision-makers still need to be convinced that basic science fundamentally depends on data to be evaluated without the expectation of immediate commercial applicability.
Table A1In the meteorological ephemerides of the Bavarian Academy of Sciences, the observers are indicated more precisely than in the ephemerides of Mannheim. Some literature with information about observers or supplementing information is indicated at the end of the table.
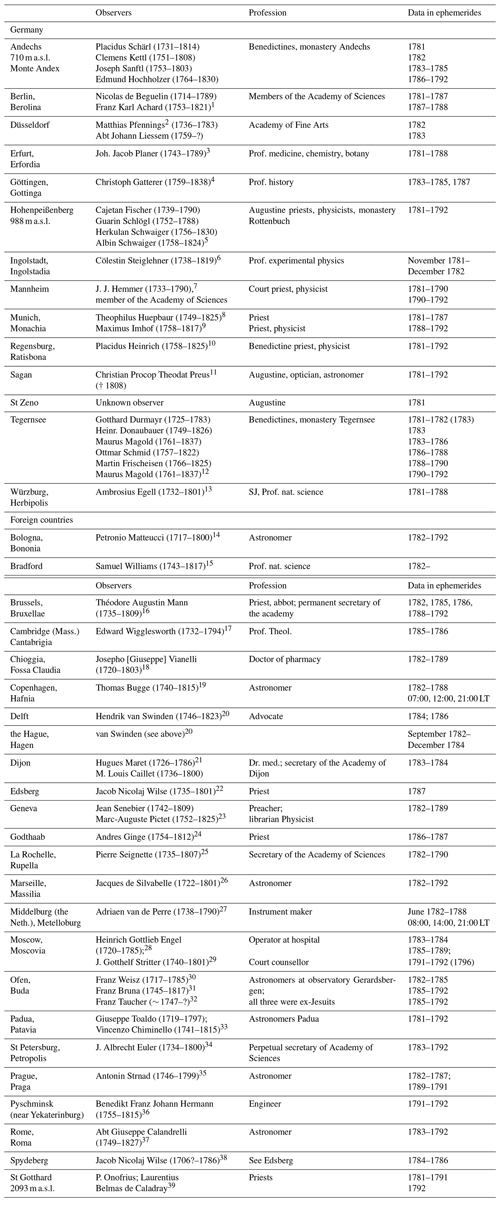
1 Allgemeine Deutsche Biographie (Oppenheim, 1875, 27–28). 2 Pfennings (Phennings) was an ex-Jesuit († 1783). Kurfürstlich Pfalzbaierischer Hof- und Staatskalender auf das Jahr 1782 (1802, p. 359). After the abolition of the Jesuits in 1773, a congregation of secular priests continued to exist in Düsseldorf. 3 Deutsch and Börngen (2010, 2011). Planer observed mostly at 06:00 or 07:00, 14:00, and 22:00 LT. 4 Heß (1878). Observations were continued in Göttingen after 1787 but not published in the Ephemerides. Neues Hannoversches Magazin 1803, Sp. 119ff. 5 Winkler (2020). 6 Heinrich (1819). 7 Stephan (2017). Hemmer had Karl König as assistant. 8 Bock (1802; Mayer and Westermayer, 1884, p. 518). 9 Bock (1802; Poggendorff, 1863). 10 Hartmann (1929). Heinrich had several assistants who helped when he was busy. 11 Leipelt (1853, p. 246). 12 Bayerische Akademie der Wissenschaften (1782–1796, 1785, Vol. 1783), Scheglmann (1908), Winkler (2020); Donaubauer seems to have assisted to Durmayr, who had become sick. 13 Hellmann (1883, p. 109). 14 Dizionario Biografico degli Italiani (2008, Vol. 72). 15 Curti et al. (1970). 16 Demarée et al. (1994; Collart, 2022). Mann had probably two assistants: Needham and Chevalier. 17 Vinovskis (1971). 18 After Garlato (1883, p. 55), Vianelli was termed as “medico et meteorologo”. 19 Bugge (1816). 20 Otto (1838, p. 430). 21 Gurlt and Hirsch (1886, Vol. 4, 1896, p. 133). 22 Wilse had sent his “Meteorographia Compendiosa” (1778) to Hemmer in 1782 (Federhofer, 2001). 23 Sigrist (2009), Cetta (2011), and Häderli et al. (2020). 24 Ginge went to Godthaab (today Nuuk) in 1782 and sent meteorological observations to Bugge in Copenhagen, who provided the first instruments (Egede, 1790, p. 307). He later obtained instruments from Hemmer and placed them in the church, and he published the results in 1786 and 1787 (Demarée and Ogilvie, 2021, p. 145). 25 Rainguet (1851, p. 552). 26 Masson (1921, 783–787). 27 Dutch instrument maker (Zuidervaart, 2006). 28 H. G. Engel was born near Halle in Saxony, Germany (Goldbeck, 1783); he had employment at many locations in Russia before he became operator at the first military hospital in Moscow in 1762. He observed at 06:00, 14:00, and 22:00 LT but in parts of 1782 at 07:00, 14:00, and 22:00 LT. 29 Poggendorff (1863). Stritter, born in Germany, was a teacher of history and deputy head at the gymnasium of the academy of St Petersburg, before he changed to Moscow in 1785, where he became conservator of the imperial archive (Friedrich, 1893). He regularly sent his observations to the Academy of Sciences in St Petersburg, as is noted in the protocols. 30 Poggendorff (1863) and Hunfalvy (1882, p. 863). 31 Poggendorff (1863). 32 Von Wurzbach (1856–1891, Vol. 43, p. 135). 33 Camuffo (2000) and Torcia (1784); Chiminello was the nephew of Toaldo. 34 Stäckel (1910). 35 Novotná and Kalvoda (2013). The observatory was situated in the Clementinum, second floor in his dwelling (von Fritsch, 1850). 36 Flügel (2006). 37 Wolfschmidt (2022). 38 Federhofer (2001). 39 Both were Capuchin monks from Milan. 40 Yaskell (2008).
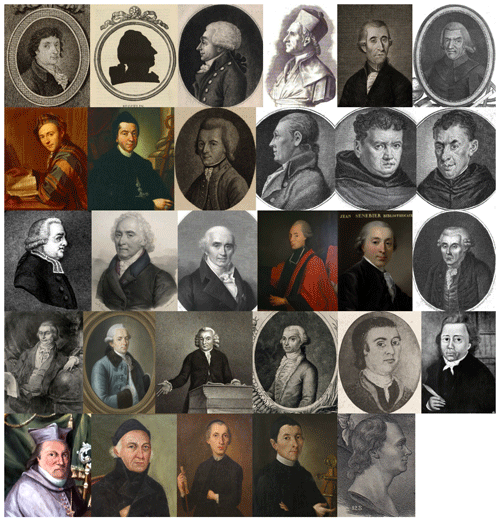
Figure A1First row (left to right): Franz Karl Achard, Berlin (a; Krünitz, 1805, Vol. 100); Nicolas de Beguelin, Berlin (b; Hoffmann, 1928); Thomas Bugge, Copenhagen (c; Allgemeine Geographische Ephemeriden, 1799); Giuseppe Calandrelli, Rome (d; De Angelis, 1845); Vincenzo Chiminello, Padua (e; courtesy of the museum La Specola, Padua); Giuseppe Toaldo, Padua (f; Toaldo, 1802); second row: Johann Albrecht Euler, St Petersburg (g; private property, Switzerland); Cölestin Steiglehner, Ingolstadt (h; courtesy Museen Regensburg; photo: Peter Ferstl); Benedikt Franz Hermann, Pyschminsk (i; Allgemeine Geographische Ephemeriden, 1805); Christoph Gatterer, Göttingen (j; Bock, 1802); Theophilus Huepbaur, Munich (k; Bock, 1802); Maximus Imhof, Munich (l; Bock, 1802); third row: Theodore Augustine Mann, Brussels (m; Colpaert, 1981); Hugues Maret, Dijon (n; Delpech, 1832); Marc-Auguste Pictet, Geneva (o; Dictionaire des Sciences naturelles, XXV, Paris, 1822); Pierre Seignette, La Rochelle (p; Source: https://www.wikidata.org/wiki/Q103981804, last access: 1 July 2023); Jean Senebier, Geneva (q; courtesy of Bibliothèque de Genève); Jacques de Silvabelle, Marseille (r; Zach, 1808); fourth row: Antonin Strnad, Prague (s; Schuster, 1931); Adriaen van de Perre, Middelburg (t; courtesy of Koninklijk Zeeuwsch Genootschap der Wetenschappen; photo: Ivo Wennekes); Hendrik van Swinden, Delft (u; Hulde aan de nagedachtenis van Jean Henri van Swinden, Amsterdam, 1824); Giuseppe Vianelli, Chioggia (v; Vianelli, 1806); Samuel Williams, Bradford (w; courtesy Haverhill Library); Jacob Nicolaj Wilse, Spydeberg (x; courtesy of Østfold fylkes billedarkiv, Norway; unknown artist); fifth row: Herkulan Schwaiger, HP (y; photo: Peter Winkler); Albin Schwaiger, HP (z1; photo: Peter Winkler); Alois Karner, Hohenpeissenberg (z2; photo: Stefan Gilge); Placidus Heinrich, Regensburg (z3; courtesy University of Regensburg, Coll. Hist. Instr.); Hendrik Nicander, Stockholm (z4; Mellin, 1847).
No datasets were used in this article. All data are publicly accessible, and the literature which was evaluated is cited in the comprehensive list of references.
The author has declared that there are no competing interests.
Publisher’s note: Copernicus Publications remains neutral with regard to jurisdictional claims in published maps and institutional affiliations.
For valuable hints and improvements to the manuscript, I am indebted to Harald Berresheim and Jost Heintzenberg. To the latter, I am additionally obliged for translating Nicander's paper written in Swedish. The possibility to reproduce the foundation charter (original in the library of the Deutscher Wetterdienst); the model of the meteorological cabinet (original in Technoseum, Mannheim); and the portraits of Elector Karl Theodor (painting in Bayerisches Nationalmuseum, Inv. no. 2009/40.1), Stephan von Stengel (Reiss-Engelhorn-Museen), and of Johann Jakob Hemmer are gratefully acknowledged. I also wish to thank the following institutions for their permission to use historical portraits of observers: Koninklijk Zeeuwsch Genootschap der Wetenschappen (Adriaen van de Perre); Museen der Stadt Regensburg (Cölestin Steiglehner); University of Regensburg, Historische Instrumentensammlung (Placidus Heinrich); museum “La Specola” and the cultural heritage manager, INAF – Astronomical Observatory of Padua (Vincenzo Chiminello); and Special Collections of the Haverhill Public Library (Samuel Williams).
This paper was edited by Kristian Schlegel and reviewed by two anonymous referees.
Allgemeine Geographische Ephemeriden, Vol. 3, Verl. d. Industrie-Comptoirs, Weimar, 1799.
Allgemeine Geographische Ephemeriden, Vol. 18 (portraits), Verl. d. Industrie-Comptoirs, Weimar, 1805.
Anonymous: Historische Nachrichten von den schrecklichen Wasser-Ergießungen von den entsetzlichen Eiß-Fahrten und von den dadurch angerichteten traurigen Verwüstungen welche in den Monaten Februar und März dieses 1784sten Jahres fast in ganz Deutschland sich eräugnet haben Aus theils öffentlichen, theils privat Nachrichten gesammelt, Scharf, 1784.
Anonymous: Johann Jakob Hemmer, Mannheimer Geschichtsblätter, 16, col. 74–80, 1915.
Aspaas, P. P. and Hansen, T. L.: The role of the Societas Meteorologica Palatina (1781–1792) in the history of Auroral research, Acta Boreal., 29, 157–176, 2012.
Bayerische Akademie der Wissenschaften: Der baierischen Akademie der Wissenschaften in München meteorologische Ephemeriden auf das Jahr 1781–1789, München, 1782–1796.
Bellani, A.: Tentativi per determinare l'aumento di volume che acquista l'acqua prima e dopo la congelazione, Giornale di Fisica, 2, 305–329, 1808.
Bernoulli, D.: Mechanik, Die gesammelten Werke der Mathematiker und Physiker der Familie Bernoulli, Bd. 3, Birkhäuser, Basel, 457 pp., ISBN 3-7643-1213-0, 1987.
Bertholon, P.: Die Elektricität der Lufterscheinungen worinne von der natürlichen Elektricität überhaupt, und von den Lufterscheinungen besonders gehandelt wird, auch die vornehmsten Phänomene der elektrischen Meteorologie nach Beobachtungen und Erfahrungen aufgestellt, De l'Electicité des Méteores Paris, 1787, Siegert, Liegnitz, 1792.
Bertucci, P.: Therapeutic Attractions: Early Applications of Electricity to the Art of Healing, in: Brain, mind and medicine: essays in eighteenth-century neuroscience, edited by: Whitaker, H. A., Springer, Berlin, 271–283, ISBN 978-0-387-70966-6, 2007.
Bock, C. W.: Sammlung von Bildnissen gelehrter Männer und Künstler, Nürnberg, 1802.
Brandes, H. W.: Dissertatio physica de repentinis variationibus in pressione atmosphaerae observatis, Staritz, Leipzig, 1826.
Braun, G.: Die Niederschlagsverhältnisse des Großherzogthums Baden, Beiträge zur Hydrographie des Grossherzogthums Baden Herausgg. von dem Centralbureau für Meteorologie und Hydrographie, Vol. 2, 1885.
Brázdil, R., Demarée, G. R., Deutsch, M., Garnier, E., Kiss, A., Luterbacher, J., Macdonald, N., Rohr, C., Dobrovolný, P., and Chromá, K.: European floods during the winter 1783/1784: scenarios of an extreme event during the “Little Ice Age”, Theor. Appl. Climatol., 100, 163–189, 2010.
Brunel, M.: Observationes phaenomenorum quorundam factae in insulis Africanis Franciae et Borbonii, Ephemerides Societatis Meteorologicae Palatinae, Observationes anni 1788, Schwann, Mannheim, 396–399, 1790.
Budde, K.: Johann Jakob Hemmer (1733–1790), in: In omnibus veritas – 250 Jahre Kurpfälzische Akademie der Wissenschaften in Mannheim, edited by: Kreutz, J., Kreutz, W., and Wiegand, H., Wellhöfer, Mannheim, 155–176, ISBN 9783954281350, 2014.
Bugge, M.: Grundzüge zu Thomas Bugges Lebensbeschreibung: dt. von Fr. Posselt, Zeitschrift für Astronomie und verwandte Wissenschaften, Vol. 2, 245–250, 1816.
Camuffo, D.: History of the long series of daily air temperature in Padova, Climate Change, 53, 7–75, 2000.
Camuffo, D., Bertolin, C., Bergonzini, A., Amore, C., and Cocheo, C.: Early hygrometric observations in Padua, Italy, from 1794 to 1826: the Chiminello goose quill hygrometer versus the de Saussure hair hygrometer, Climatic Change, 122, 217–227, 2014.
Camuffo, D., Bertolin, C., Craievich, A., Granziero, R., and Enzi, S.: When the Lagoon was frozen over in Venice from A.D. 604 to 2012: evidence from written documentary sources, visual arts and instrumental readings, Méditerranée, https://doi.org/10.4000/mediterranee.7983, 2017.
Camuffo, D., della Valle, A. , Becherini, F., and Zanini, V.: Three centuries of daily precipitation in Padua, Italy, 1713–2018: history, relocations, gaps, homogeneity and raw data, Climatic Change, 162, 923–942, 2020.
Cappel, A.: Phänologische Beobachtungen der Societas Meteorologica Palatina, Promet, 10, 52–53, 1980a.
Cappel, A.: Societas Meteorologica Palatina (1780–1795), Annalen der Meteorologie (N. F.), 16, 11–27, 1980b.
Cappel, A.: Societas meteorologica Palatina (1780–1795), Beil. z. Mitt.-Bl. Deutscher Wetterdienst, 11, 1–16, 1980c.
Cassidy, D. C.: Meterology in Mannheim: The Palatine Meterological Society, 1780–1795, Sudhoffs Archiv für Geschichte der Medizin und der Naturwissenschaften, 69, 8–25, 1985.
Celoria, G.: Sulle variazioni periodiche e non periodiche della temperatura nel clima di Milano, Hoepli, Milano, 1874.
Cetta, T.: Senebier, Jean, Historisches Lexikon Schweiz, 11, p. 436, ISBN 978-3-7965-1910-9, 2011.
Chenoweth, M.: The U.S. Army Medical Department's Involvement in the Early History of Weather Observing: Was Benjamin Waterhouse the First Army Weather Observer?, B. Am. Meteorol. Soc., 77, 559–563, 1996.
Cocheo, C. and Camuffo, D.: L'igrometro di Chiminello e le misure igrometriche effettuate a Padova per la 'Meteorologica Societas Palatina', Mannheim, Centro per la Storia dell'Università di Padova e Osservatorio Astronomico di Padova, Padova, 743–762, 2000.
Collart, M.: Théodore Mann, savoir et pouvoir, Académie royale de Belgique, Bruxelles, ISBN 978-2-8031-0863-3, 2022.
Colpaert, H.: Th. A. Mann (1735–1809) (portrait), dissertation, University Leuven, 1981.
Cotte, L.: Recherches météorologiques, Observations sur la Physique, XLI, 269–283, 1792.
Cotte, L.: Mémoire sur la période lunaire de dix-neuf ans, Paris, 1805.
Csernus-Molnár, I. and Kiss, A.: A XVIII. század végi magyarországi műszeres meteorológiai mérések feldolgozási és vizsgálati lehetőségei: (Research and study possibilities of late 18th-century instrumental weather measurement series in Hungary), Conference Környezettörténet, 2, 203–214, ISBN 978-615-5086-03-8, 2011.
Csernus-Molnár, I., Kiss, A., and Pócsik, E.: 18th-Century Daily Measurements and Weather Observations in the Se-Carpathian Basin: A Preliminary Analysis of the Timişoara Series (1780–1803), Journal of Environmental Geography, 7, 1–9, 2014.
Cubasch, U. and Kadow, C.: Global Climate Change and Aspects of Regional Climate Change in the Berlin-Brandenburg Region, DIE ERDE – Journal of the Geographical Society of Berlin, 142, 3–20, 2011.
Curti, M., Tillman, W., and Williams, S.: Philosophical Lectures by Samuel Williams, LL. D., on the Constitution, Duty, and Religion of Man, T. Am. Philos. Soc., 60, 1–130, https://doi.org/10.2307/1005975, 1970.
Daniell, J. F.: Essays and Observations: Vol. 2, Underwood, London, 1827.
De Angelis, G.: L'Abbate Giuseppe Calandrelli, L'album: giornale letterario e di belle arti, 12, 137–141, 1845.
de Lamarck, J.-B.: De l'influence de la lune sur l'atmosphère terrestre, Paris, 1798.
Delpech, F. S.: Iconographie des contemporains depuis 1789 jusqu'à 1829 (portrait Maret), Vol. 2, Delpech, Paris, 1832.
Demarée, G. R. and Chuine, I.: A concise history of the phenological observations at the Royal Meteorological Institute of Belgium, Proceedings of the HAICTA-2006 Conference, 20–23 September 2006, Volos, Greece, Vol. III, Phenology-Agrometeorology, 815–824, 2006.
Demarée, G. R. and Ogilvie, A.: Early Meteorological Observations in Greenland: The Contributions of David Cranz, Christian Gottlieb Kratzenstein and Christopher Brasen, in: Legacies of David Cranz's 'Historie von Grönland' (1765), edited by: Jensz, F. and Petterson, C., Palgrave, Macmillan, 141–164, ISBN 978-3-030-63997-6, 2021.
Demarée, G. R., van Engelen, A. E. V., and Geurts, H. A. M.: Les observations météorologiques de Théodore-Augustin Mann, Ciel et Terre, 110, 41–48, 1994.
Descartes, R.: Discours de la méthode pour bien conduire sa raison, & chercher la verité dans les sciences. Plus La dioptrique, les météores et la géométrie, qui sont des essais de cete méthode, Leyden, 1637.
Destombes, M.: Mappemondes, Israel, Amsterdam, 1964.
Deutsch, M. and Börngen, M.: Johann Jacob Planer (1743–1789) und sein meteorologisches Wirken im Rahmen der Societas Meteorologica Palatina, in: Universitäten und Akademien, edited by: Kästner, I. and Kiefer J., Shaker, Aachen, 211–224, ISBN 9783832290498, 2010.
Deutsch, M. and Börngen, M.: Anmerkungen zum Leben des Erfurter Arztes und Naturwissenschaftlers Johann Jacob Planer, Mitteilungen des Vereins für die Geschichte und Altertumskunde von Erfurt, 72, 51–69, 2011.
Dizionario Biografico degli Italiani, Vol. 72, Istituto della Enciclopedia Italiana, 2008.
Dove, H. W.: Über das Gesetz der Stürme, Reimer, Berlin, 1857.
Egede, P. H.: Nachrichten von Grönland, Proft, Kopenhagen, 1790.
Ellinger, A.: Beyträge über den Einfluß der Himmelskörper auf unsere Atmosphäre, Fleischmann, München, 1814.
Ernesti, J. A.: Neue theologische Bibliothek, darinnen von den neuesten theologischen Büchern und Schriften Nachricht gegeben wird, Bd. 3.1, Breitkopf, Leipzig, 1773.
Federhofer, M.-T.: Wetterzeichen. Zum meteorologischen Codierungsverfahren Jacob Nicolaj Wilses (1735–1801), Cardanus, 2, 13–30, 2001.
Federhofer, M.-T.: “Magnetische Ungewitter” und “Erd-Lichter”: Alexander von Humboldt und das Nordlicht, Z. Germanistik, 24, 267–281, 2014.
Fleming, J. R.: History of meteorology, A History of modern Science and Mathematics, Scribner & Sons, 3, 184–217, ISBN 0684806363, 2002.
Flügel, H. W.: Das abenteuerliche Leben des Benedikt Hermann (1755–1815); vom steirischen Bauernsohn zum Chevalier und Intendanten der russischen Bergwerke, Böhlau, Wien, 334 pp., 2006.
Frångsmyr, T., Heilbron, J. L., and Rider, R. E.: The Quantifying Spirit in the Eighteenth Century, Univ. of Calif. Pr, Berkeley, https://doi.org/10.1525/9780520321595, 1990.
Friedrich, O.: Stritter, Johann Gotthelf von, in: Allgemeine Deutsche Biographie, Duncker & Humblot, 36, p. 598, 1893.
Fritz, H.: Die geographische Verbreitung des Polarlichtes, Petermanns Georgr. Mitt., 20, 347–358, 1874.
Fuchs, P.: Palatinatus illustratus: die historische Forschung an der kurpfälzischen Akademie der Wissenschaften, Bibliograph. Inst, Mannheim, 1963.
Galilei, G.: Il saggiatore, Roma, 1623.
Garlato, A.: Canti del Popolo di Chioggia, Naratovich, Venezia, 1883.
Gatterer, J. C.: De anno meteorologico fundamentali, Commentationes der Ges. der Naturwiss. Göttingen, Dieterich, III, 82–120, 1780.
Gisler, O.: Das Wetter zu Ende des 18. Jahrhunderts, Geogr. Helv., 40, 205–222, https://doi.org/10.5194/gh-40-205-1985, 1985.
Goldbeck, J. F.: Litterarische Nachrichten von Preußen: Tl. 2, Selbstverlag, Leipzig, Dessau, 1783.
Gurlt, E. and Hirsch, A.: Biographisches Lexikon der hervorragenden Ärzte aller Zeiten und Völker, Urban & Schwarzenberg, München, 1886.
Häderli, S., Pfister, S., Villinger, L., Brugnara, J., and Brönnimann, S.: Two meteorological series from Geneva, 1782–1791, Geographia Bernensia, 96, 33–46, 2020.
Hartmann, L.: Der Physiker und Astronom P. Placidus Heinrich von St. Emmeram in Regensburg (1758–1825): Seine wissenschaftlichen Arbeiten und sein handschriftlicher Nachlass, STMBO, 16, 157–182, 316–351, 1929.
Hattori, K., Hayakawa, H., Ebihara, Y.: The extreme space weather events in October 1788, Publ. Astron. Soc. Jpn., 73, 1367–1374, 2021.
Haustein, H.-D.: Quellen der Messkunst, Berlin, New York, ISBN 9783110178333, 2004.
Heinrich, P.: Oscillationes Mercurii in tubo torricelliano ingruentibus procellis et tempestatibus observatae in museo physico Ratisbonae ad St. Emmeramum annis 1788 et 1789, München, 1794.
Heinrich, P.: Vom Gefälle der Donau: Schreiben an Herrn von Humboldt, vom Professor Heinrich, Zachs Monatl. Correspondenz, 25, 368–379, 1812.
Heinrich, P.: Die Temperatur von St. Petersburg aus einer Reihe zwanzigjähriger Beobachtungen hergeleitet, Journal für Chemie und Physik, 8, Beilage 3, 1–21, Beilage 4, 1–14, 1813.
Heinrich, P.: Kurze Lebensgeschichte des letzten Fürst-Abtes zu St. Emmeram in Regensburg Cölestin Steiglehner, Daisenberger, Regensburg, VI, 107 pp., 1819.
Hell, M.: Maximilian Hells Schreiben über die in Wien entdekte Magnet-Cur an einen seiner Freunde, Neueste Nachrichten aus Wien von den vermittelst des Magnets geschehen seyn sollenden Curen, 12.01.1775, 11–15, 1775.
Hellman, G.: The Laki volcanic eruption of 1783–1784 a reappraisal and reinterpretation of the consequences of the event in Europe. Villain or fall guy?, Thesis, History, p. 80, Rennes, 2021.
Hellmann, G.: Repertorium der deutschen Meteorologie, Engelmann, Leipzig, 1883.
Hellmann, G.: Die Vorläufer der Societas Meteorologica Palatina, Beiträge zur Geschichte der Meteorologie, 5, 139–147, 1914.
Hemmer, J. J.: Kritische Anmerkungen, Rheinische Beiträge zur Gelehrsamkeit, Kurfürstl. neue Hof- und akademische Buchhandlung, 457–470, 1779.
Hemmer, J. J.: Descriptio Instrumentorum Societatis Meteorologicae Palatinae tam eorum, quæ observatoribus suis distribuit quam quibus praeter haec Manheimii utitur, Officinae Novae Societas Typographcae Electoralis, Manheimii, 1 volume, 1782a.
Hemmer, J. J.: Letter to Steigenberger May 18th, 1782, Bavar. States Library, Cgm 3210, fol 10, 1782b.
Hemmer, J. J.: [Untitled translation of the speech held in German], Ephemerides Societatis Meteorologicae Palatinae, Historia et Observationes anni 1781, Schwann, Mannheim, 18–23, 1783.
Hemmer, J. J.: Urtheil der Gesellschaft der Wissenschaften zu Mannheim über die eingelaufenen Preisschriften, von Erfindung eines vergleichbaren Feuchtigkeitsmessers in ihrer öffentlichen, den 20. Weinmonat 1783 gehaltenen Versammlung, abgelesen vom Herrn geistlichen Rath Hemmer, Pfälzisches Museum, 1, 388–410, 1783/1784.
Hemmer, J. J.: Vaporis a. 1783 succincta Historia, Societatis meteorologicae Palatinae, Observationes anni 1783, Schwann, Mannheim, 57–60, 1785.
Hemmer, J. J.: Nachricht, von der kurpfälzischen Witterungsgesellschaft, Pfalzbaierisches Museum, 3, 259–278, 1785/1786.
Hemmer, J. J.: Merkwürdige Erscheinung an einer vorüber ziehenden Wolke, mit Anmerkungen begleitet, Historia et commentationes Academiae Electoralis Scientiarum, VI, 319–324, 1790.
Hemmer, J. J.: Von der Variabilität der Magnetnadel zur Zeit des Nordlichts, Grens Journal der Physik, VIII, 88–90, 1792.
Heß, R.: Gatterer, Christoph, Allgemeine Deutsche Biographie, 8, 409–410, 1878.
Hildebrandsson, H. H. and Teisserance de Bort: Recherches ancienne sur les tempêtes des zones tempérées. Théories centripèdes de Brandes, Espy et Loomis, Les bases de la météorologie dynamique, historique, Gauthier-Villars et Fils, 1, 45–60, 1898.
Hochadel, O.: “In nebula nebulorum”: The Dry Fog of the Summer of 1783 and the Introduction of Lightning Rods in the German Empire, T. Am. Philos. Soc., 99, 45–70, 2009.
Hoffmann, P.: Schattenrisse sechs Berlinscher Gelehrten. In Holz geschnitten von J. F. G. Unger, dem Jüngern, Berlin 1779, Reichsdruckerei, Berlin, 1928.
Hollmann, S.: Nöthiger Unterricht von Barometern und Thermometern, Grape, Göttingen, 1783.
Holm, S. M.: De incendio terrae in Islandia, Societatis meteorologicae Palatinae. Observationes anni 1783, Schwann, Mannheim, 689–694, 1785.
Holopainen, J.: Reconstruction of past climates from documentary and natural sources in Finland since the 18th century, Diss. Dept. Geology Uni. Helsinki, Helsinki, 2005.
Holyoke, E. A.: An Estimate of the Excels of the Heat and Cold of the American Atmosphere beyond the European, in the Same Parallel of Latitude: To Which Are Added, Some Thoughts on the Causes of This Excels, Memoirs of the American Academy of Arts and Sciences, American Academy of Arts & Sciences, 2, 65–88, 1793.
Hunfalvy, P.: Ungarische Revue, Kilian, Budapest, 1882.
Ihne, E.: Geschichte der pflanzenphänologischen Beobachtungen in Europa, Beiträge zur Phänologie, Ricker, Göttingen, 1–105, 1894.
Jefferson, T.: The papers of Thomas Jefferson, 21, Princeton Univ. Press, Princeton, NJ, 592 pp., ISBN 0-8071-1037-X, 1983.
Kalela-Brundin, M.: The narrow ring of 1784 in tree-ring series of Scots pine (pinus sylvestris L.) in southwest Norway – a possible result of volcanic eruptions in Iceland, in: Holocene tree line oscillations, dendrochronology and palaeoclimate, edited by: Frenzel, B., Fischer, Stuttgart u.a., 105–118, ISBN 3-437-25386-7, 1996.
Kämtz, F. L.: Lehrbuch der Meteorologie, Vol. 1, Gebauer, Halle, 1831.
Kämtz, F. L.: Lehrbuch der Meteorologie, Vol. 2, Gebauer, Halle, 1832.
Kämtz, F. L.: Lehrbuch der Meteorologie, Vol. 3, Gebauer, Halle, 1836.
Karner, A. G.: Altitudines, Massiliae, Manheimii, Ratisbonae, Monachii, St. Andex, Tegernsee, Peißenberg et montis St. Gotthardi supra libellam maris mediterranei ope barometricarum et thermometricarum obseruationum determinatae, Denkschriften k. Bay. Akademie der Wiss., 3, 14, 437–448, 1812.
Kington, J.: The weather of the 1780s over Europe, Cambridge Univ. Press, Cambridge, X, 164 pp., ISBN 0521260795, 1988.
Kistner, A.: Die Pflege der Naturwissenschaften in Mannheim zur Zeit Karl Theodors: Geschichte der kurpfälzischen Akademie der Wissenschaften in Mannheim, Altertumsverein, Mannheim, 1930.
Kleinert, A.: Der messende Luchs, NTM – Zeitschrift für Wissenschaften, Technik und Medizin, 17, 177–206, 2009.
Koenig, C.: Observationes circa varorem aestivum a. 1783 in objectis coelestibus factae. Societatis meteorologicae Palatinae. Observationes anni 1783,, Schwann, Mannheim, 60–61, 1785.
Korte, M., Mandea, M., and Matzka, J.: A historical declination curve for Munich from different data sources, Phys. Earth Planet. In., 177, 161–172, 2009.
Kratzenstein, C. G.: Abhandlung von dem Einfluß des Mondes in die Witterungen und in den menschlichen Körper, Hemmerde, S. L., Halle, 1747.
Krause, J. T.: Ultrasonic measurement technique to study thermometric effects in glass, J. Non-Cryst. Solids, 14, 32–40, 1974.
Kreutz, J.: Niedergang und Ende der Akademie 1790–1806, in: In omnibus veritas – 250 Jahre Kurpfälzische Akademie der Wissenschaften in Mannheim, edited by: Kreutz, J., Kreutz, W., and Wiegand, H., Wellhöfer, Mannheim, 205–220, ISBN 9783954281350, 2014.
Krünitz, J. G.: Ökonomisch-technologische Encyklopädie, [portrait before title page], Vol. 100, Pauli, Berlin, 1805.
Kurfürstlich Pfalzbaierischer Hof- und Staatskalender auf das Jahr 1782, Franz, München, p. 247, 1802.
Lalande, J: Bibliographie astronomique 1781–1802, Paris, 1803.
Lamb, H. H.: Historic storms of the North Sea, British Isles and Northwest Europe, Cambridge Univ. Press, Cambridge, 204 pp., ISBN 0521375223, 1991.
Lambert, J. H.: Entwurf eines akademischen Systems in seinen Theilen, und deren Verbindungen; dann eines dreyfachen Tagregisters, vom Profeßor Lambert 1761, edited by: Westenrieder, L., Geschichte der bayerischen Akademie der Wissenschaften, Bd. 1, 1784, 482–531, 1761.
Lambert, J. H.: Von der Meteorologie, Akademischer Kalender, Anhang. Augsburg, 1762.
Lambert, J. H.: Abhandlung Von den Barometerhöhen und ihren Veränderungen, Abh. der k. Bay. Akademie d. Wiss., 3, 75–182, 1765.
Lambert, J. H.: Exposé de quelques Observations qu'on pourrait faire en météorologie: Année 1771, Nouveaux Mémoires de l'Academie Royale des Sciences de Berlin, Haude & Spener, Berlin, 60–65, 1773.
Lamont, J.: Beobachtungen des Meteorologischen Observatoriums auf dem Hohenpeißenberg von 1793–1850, Annalen der K. Sternwarte in München, Supplementband III, 1–787, 1851.
Leipelt, A.: Geschichte der Stadt und des Herzogthums Sagan, Rauert, Sorau, 1853.
Libri, G.: Memoire sur la determination de l'ècheel du thermomètre de l'Académie del Chimento, Anales de Chimie et de Physique, 45, 354–361, 1830.
Lichtenberg, G. C.: Briefwechsel, Beck, München, XI, Beck, ISBN 3406094023, 1985.
Lüdecke, C.: Von der Kanoldsammlung (1717–1726) zu den Ephemeriden der Societas Meteorologica Palatina (1781–1792). Meteorologische Quellen zur Umweltgeschichte des 18. Jahrhunderts, Cottbuser Studien zur Geschichte von Technik, Arbeit und Umwelt, Waxmann, Münster, München u.a., 30, 97–119, ISBN 978-3-8309-6904-4, 2010.
Malavois, C.: Epitome observationum meteorologicarum factarum in urbe Asiatica Puhnah, quae marattarum metropolis est, Societatis meteorologicae Palatinae, Observationes anni 1788, Schwann, Mannheim, p. 400, 1790.
Maret, H.: Dissertatio de nebula in mensium Junii Juliique a. 1783 decursu Divione observata, Societatis meteorologicae Palatinae, Observationes anni 1783, Schwann, Mannheim, 468–472, 1785.
Masson, P.: Encyclopédie des Bouches-du-Rhône, Bd. III, Archives départementales, Archives Dép. des Bouches-du-Rhone, Marseille, 1921.
Mayer, A. and Westermayer, G.: Statistische Beschreibung des Erzbisthums München-Freising, Manz, München, 1884.
Mayr, G. K.: Sammlung der Kurpfalz-Baierischen allgemeinen und besonderen Landes-Verordnungen, Vol. 4, München, 1788.
McNally, L. K.: The weather of 1785, Diss. Univ. Maine, 2004.
Meichle, F.: Seegefrörne und Eisprozession in Vergangenheit und Gegenwart, Schriften des Vereins für Geschichte des Bodensees und seiner Umgebung, 81, 145–170, 1963.
Mellin, G. A.: 427 porträtter af namnkunniga svenske män och fruntimmer, Berg, Stockholm, 1847.
Meyer d. J., J. R.: Systematische Darstellung aller Erfahrungen in der Naturlehre, Sauerländer, Aarau, 1806–1808.
Middleton, W. E. K.: The history of the barometer, Johns Hopkins Press, Baltimore, 1964.
Moberg, A., Bergström, H., Krigsman, J. R., and Svanered, O.: Daily air temperature and pressure series for Stockholm (1756–1998), Climatic Change, 53, 171–212, 2002.
Müller, W.: Universität und Orden, Dunker & Humblot, Berlin, ISBN 3-428-06135-7, 1986.
Müller, W.: Der Jesuitenorden und die Aufklärung im süddeutsch-österreichischen Raum, in: Katholische Aufklärung – Aufklärung im katholischen Deutschland, edited by: Klueting, H., Meiner, Hamburg, ISSN 0044-2364, 1993.
Nicander, H.: Om Tabell-Vårkets Tillstånd, ifrån 1772 till 1795, Kongl. Vetenskaps Akademiens nya Handlingar, XII, 298–334, 1801.
Nollet, J. A.: Lettres sur l'Électricité, Guérin, Paris, 1753.
Novontá, E. and Kalvoda, J.: Antonin Strnad (1746–1799) – the first professor of physical geography at Charles University in Prague, AUC Geographica, 48, 41–46, 2013.
Oppenheim, A.: Achard, Franz Karl, Allgemeine Deutsche Biographie, Duncker & Humblot, Berlin, Vol. 1, 27–28, 1875.
Otto, F. J.: Die Gesammtliteratur Niederlands, oder Leben und Wirken der holländischen Schriftsteller seit dem dreizehnten Jahrhundert bis auf unsere Zeit, Bibliograph. Institut, Hildburghausen, 1838.
Pappert, D., Brugnara, Y., Jourdain, S., Pospieszyńska, A., Przybylak, R., Rohr, C., and Brönnimann, S.: Unlocking weather observations from the Societas Meteorologica Palatina (1781–1792), Clim. Past, 17, 2361–2379, https://doi.org/10.5194/cp-17-2361-2021, 2021.
Pelz, J.: Eine kritische Betrachtung zur Geschichte der Temperaturmessungen und deren Auswertung in Berlin seit 1701, Meteorol. Anhandl. Fr. Univ. Berlin, 4.4, Berlin, 1993.
Pilgram, A.: Anton Pilgrams Untersuchungen über das Wahrscheinliche der Wetterkunde durch Vieljährige Beobachtungen, von Kurzbeck, Wien, [12] Bl., 608 S., [2] Bl., [1] gef. Bl., von Kurzbeck, 1788.
Poey, A.: Action of electricity on the germination and growth of plants, Report of the Commissioner of Agriculture, 1869, Government Printing Office, 152–157, 1879.
Poggendorff, J. C.: Biographisch-literarisches Handwörterbuch der exakten Naturwissenschaften: enthaltend Nachweisungen über Lebensverhältnisse und Leistungen von Mathematikern, Astronomen, Physikern, Chemikern, Mineralogen, Geologen usw. aller Völker und Zeiten, Barth, Leipzig, 1863.
Priestley, J.: The History and Present State of Electricity: With Original Experiments, Dodsley, Johnson and Davenport, Cadell, London, 1767.
Raicich, F.: Long-term variability of storm surge frequency in the Venice Lagoon: an update thanks to 18th century sea level observations, Nat. Hazards Earth Syst. Sci., 15, 527–535, https://doi.org/10.5194/nhess-15-527-2015, 2015.
Rainguet, P.-D.: Biographie saintongeaise, Niox, Saintes, 1851.
Reich, K.: Der Humboldt’sche Magnetische Verein im historischen Kontext, HiN – Alexander Von Humboldt Im Netz, Internationale Zeitschrift für Humboldt-Studien, 24, 53–74, https://doi.org/10.18443/357, 2023.
Retz, N.: Herrn Retz's, der Arzneywissenschaft Doktors, Abhandlung vom Einfluße der Witterung auf die Arzneywissenschaft und den Ackerbau nebst der Beschreibung eines neuen vergleichbaren Hygrometers. Eine gekrönte Preisschrift, Originalpublikation 1779, Henning, Greiz, [1] Bl., 210 pp., [1] Bl., 1786.
Rigby, M.: The evolution of international cooperation in meteorology, B. Am. Meteorol. Soc., 46, 630–633, 1965.
Ripperger, A.: Die Influenza, Lehmann, München, 1892.
Rosenthal, G. E.: Briefe an Graf Brocke, Buchhandl. d. Gelehrten, Leipzig/Nordhausen, 1784/1785.
Scheglmann, A. M.: Geschichte der Säkularisation im rechtsrheinischen Bayern, Habbel, Regensburg, VIII, 820 pp., 1908.
Schelling, F. W.: Über das sogenannte Wetterschießen, Sämtliche Werke, Cotta, Stuttgart, 8, 427–438, 1861.
Schlögl, G.: Tabvlae Pro Redvctione Quorvmvis Statvvm Barometri Ad Normalem Qvemdam Caloris Gradvm, Lindaver, Monachii, Ingolstadii, [4] Bl., XXX, 122 pp., [3] Bl., 1787.
Schön, J.: Die Witterungskunde in ihrer Grundlage: Mit 1 Titelkupf., 1 Chärtch. u. 12 lith. Tab, Dümmler, Würzburg, 1818.
Schuster, F.: Život a dílo astronom a Antonína Strnada, Rocnic, 12, 79–115, 1931.
Schwaiger, A.: Descriptio Atmidometri (Evaporatorii) nostri & Methodi quam in eo observando adhibemus, Ephemeriden der Societas Meteorologica Palatina, Schwann, Mannheim, p. 300, 1783.
Schwaiger, A.: Versuch einer meteorologischen Beschreibung des hohen Peißenbergs als eine nöthige Beylage zu dessen Prospektskarte, Enth.: Prospects Charte von Hochenpeissenberg, Franz, München, 1791.
Schweingruber, F. H., Briffa, K. R., and Jones, P. D.: Yearly maps of summer temperatures in Western Europe from A.D. 1750 to 1795 and Western North America from 1600 to 1982: Results of radiodensitometrical study of tree rings, Vegetatio, 92, 5–71, 1991.
Seiferheld, G. H.: Electrischer Versuch wodurch Wassertropfen in Hagelkörner verändert worden samt der Frage an die Naturforscher: Ist eine Hagelableitung ausführbar, und Wie?, Monath, Altdorf, Nürnberg, [3] Bl., 38 pp., 1790.
Senebier, J.: Dissertatio de vapore Generae observato durante a. 1783, Societatis meteorologicae Palatinae, Observationes anni 1783, Schwann, Mannheim, 431–435, 1785.
Sheynin, O. B.: On the History of the Statistical Method in Meteorology, Arch. Hist. Exact Sci., 31, 53–95, 1984.
Sigrist, R.: Pictet, Marc-Auguste, Historisches Lexikon Schweiz, 9, 729–730, ALS97156, 2009.
Stäckel, P.: Johann Albrecht Euler, Vierteljahrsschrift der Naturforschenden Gesellschaft in Zürich NGZH, 55, 63–75, 1910.
Steiglehner, C.: Atmosphaerae pressio varia observationibus baroscopicis propriis et alienis, Haberberger, Ingolstadii, [4] Bl., 58 pp., [8] Bl., [5] gef. Bl., 1783.
Stephan, F. J.: Johann Jakob Hemmer: 1773–1790 kurfürstlicher Hofkaplan in Mannheim, Sprachreformer, Physiker und Meteorologe ein Gelehrter in praktischer Absicht Lebensbeschreibung aus der Sicht eines Familienforschers, Cardamina Verlag, Weißenthurm, 292 pp., ISBN 3864243696, 2017.
Stoll, J. J.: Beleuchtung einiger Vorurtheile in Ansehung der Donnerwetter und Blizableiter, Selbstverl., Lindau, 54 pp., 1790.
Strnadt, A.: Beyträge zu der Geschichte des Winters im Christmonaste 1788, Sammlung Physikalischer Aufsätze, besonders die Böhmische Naturgeschichte betreffend, 3, 40–88, 1793.
Toaldo, G.: Essai de météorologie appliquée à l'Agriculture (Prix de la Société Royale des Sciences de Montpellier, en 1774, Observations sur la Physique, X, 249–279, 1777a.
Toaldo, G.: Witterungslehre für den Feldbau: Eine, von der Königl. Societät der Wissenschaften zu Montpellier gekrönte Preisschrift, über die für das Jahr 1774. aufgelegte Frage: Was hat die Witterung für Einfluß auf den Wachsthum der Pflanzen, und was für praktische Folgerungen lassen sich, in Rücksicht auf den Wachsthum, aus den verschiedenen bisher gemachten Wetterbeobachtungen ziehen?, Himburg, Berlin, 1777b.
Toaldo, G.: Completta Raccolta, Tom. 1, Andreola, Venezia, 1802.
Torcia, M.: Briefe des Herrn Michael Torcia an den Herrn Professor Toaldo zu Padua von dem Höhenrauch des vergangenen Jahres zu Neapel und in Calabrien, Der Teutsche Mercur, Verl. der Ges., Weimar , 3–16, 1784.
Traumüller, F.: Die Mannheimer meteorologische Gesellschaft 1780–1799, Dürr, Leipzig, 1885.
Van Swinden, H.: Observationes nebulam quae mense Junio 1783 apparuit spectantes, Societatis meteorologicae Palatinae, Observationes anni 1783, Schwann, Mannheim, 678–688, 1785.
Vianelli, G. V.: La Marina ed altre poesie pescatorie di Giuseppe Valentino Vianelli di Chioggia socio di varie accademie, premessovi il di lui elogio, Zerletti, Venezia, 1806.
Vinovskis, M. A.: The 1789 Life Table of Edward Wigglesworth, J. Econ. Hist., 31, 570–590, 1971.
Volney, C.-F.: Oeuvres complètes de C.-F. Volney, Comte et Pair de France, membre de l'Académie Française, honoraire de la Société Asiatique, séante à Calcutta, Vol. 7, 1–497, Bossange, Paris, 1821.
von Felbiger, J. I.: Anleitung, jede Art der Witterung genau zu beobachten, in Karten zu verzeichnen, zu vergleichen, und daraus, besonders für die Landwirthschaft, nützliche Folgen zu verzeichnen, Lauhen, Sagan, 1–92, 1773.
von Fritsch, C.: Grundzüge einer Meteorologie für den Horizont von Prag, entworfen aus den an der k. k. Universitäts-Sternwarte daselbst in den Jahren 1771 bis 1846 angestellten Beobachtungen: Aus den Abh. d. k. böhm. Ges. d. Wiss. V Folge, VII Bd., bes. abgedruckt, Gottl. Haase, Prag, 1850.
von Grafenstein, U., Erlenkeuser, H., Müller, J., Trimborn, P., and Alefs, J.: A 200-year mid-European air temperature record preserved in lake sediments: An extension of the δ18Op-air temperature relation into the past, Geochimica et Cosmochimica Acta, 60, 4025–4036, 1996.
von Humboldt, A.: Des lignes isothermes et de la distribution de la chaleur sur le globe, par Alexandre de Humboldt, Mém. Soc. d'Arcueil, 3, 462–602, 1817.
von Humboldt, A.: Examen critique de l'histoire de la géographie du nouveau continent et des progrès de l'astronomie nautique aux quinzième et seizième siècles, Librairie de Gide, Paris, 247 pp., 1837.
von Martius, C. F. P.: Erinnerung an Mitglieder der mathematisch-physikalischen Classe der K. Bayr. Akademie der Wissenschaften eine Rede, vorgetragen in der öffentlichen Sitzung zur Feier des akademischen Saecularfestes am 29. März 1859, Fest- und Gedächtnisreden/Bayerische Akademie der Wissenschaften, München, 74 pp., 1859.
von Rudloff, H.: Die Schwankungen und Pendelungen des Klimas in Europa seit dem Beginn der regelmäßigen Instrumenten-Beobachtungen (1670), Die Wissenschaft, Vieweg, Braunschweig, 1967.
von Stengel, S.: Philosophische Betrachtungen über die Alpen, Rede am Stiftungstag der bay. Akad. Wiss. am Stiftungstage 28. März 1786 vorgetragen, Franz, München, 1786.
von Stengel, S. and Ebersold, G.: Denkwürdigkeiten, Schriften der Gesellschaft der Freunde Mannheims und der Ehemaligen Kurpfalz, Mannheimer Altertumsverein von 1859, 23, Palatium-Verl., Mannheim, VII, 1–170, ISBN 3-920671-06-6, 1993.
von Wurzbach, C.: Biographisches Lexikon des Kaiserthums Oesterreich: mehrbänd. Werk, Wien, 1856–1891.
von Yelin, J. C.: Über die Veränderlichkeit des Nullpunktes in den Quecksilber und Weingeist-Thermometern, Kastners Archiv für die gesamte Naturlehre, 3, 109–122, 1824.
Voss, J.: Die Kurpfälzische Akademie der Wissenschaften und ihre Beziehungen zu Frankreich 1763–1800, Mannheimer Geschichtsblätter N.F., 1, 181–194, 1994.
Wilse, J. N.: Meteorographia Compendiosa eller en kort Maade, ved beqvemme Tegn, at optegne alle slags Veyr, veyrskifter og Luftsyner, Godiche, Kopenhagen, 1778.
Wilse, J. N.: Forsøg tilstedsvarende Meteorologisk Natur- og Huusholdrings-Calender for Norge Søndenfields, Christiania, 1780.
Winkler, P.: Early observations of and knowledge on air electricity and magnetism at Hohenpeissenberg during the Palatina era, Algorismus, 52, 55–68, 2005.
Winkler, P.: Revision and necessary correction of the long-term temperature series of Hohenpeissenberg, 1781–2006, Theor. Appl. Climatol., 98, 259–268, 2009a.
Winkler, P.: Wissenschaftshistorische Untersuchungen zur Geschichte und insbesondere zur Datenqualität der langen meteorologischen Reihen des Observatoriums Hohenpeißenberg, Berichte des DWD, 233, 1–187, ISBN 978-3-88148-442-8, 2009b.
Winkler, P.: Untersuchung zu historischen und neuzeitlichen Dampfdruckmessungen am Observatorium Hohenpeißenberg, Ber. DWD, 1–206, ISBN 978-3-88148-479-4, 2015.
Winkler, P.: Georg Friedrich Branders Briefe an das Kloster Polling 1754–1780, Algorismus, Studien zur Geschichte der Mathematik und der Naturwissenschaften, edited by: Folkerts, M., 1–358, ISBN 3-936905-63-0, 2017.
Winkler, P.: Geschichte der Meteorologie in Bayern bis zum Jahr 1900, Geschichte der Meteorologie in Deutschland, Deutscher Wetterdienst, Langen, 1–236, ISBN 978-3-88148-520-3, 2020.
Wolf, R.: Mitteilung über die Sonnenflecken, Vierteljahresschrift Nat.-forsch Ges. Zürich, 6, 416–451, 1861.
Wolf, R.: Über die elfjährige Periode in den Sonnenflecken und erdmagnetischen Variationen, Poggendorffs Annalen der Physik, CXVII, 502–509, 1862.
Wolff, C.: Vollständiges mathematisches Lexikon, Gleditsch, Leipzig, 1716.
Wolfschmidt, G.: Astronomy in Culture – Cultures of Astronomy. Astronomie in der Kultur – Kulturen der Astronomie: Featuring the Proceedings of the Splinter Meeting at the Annual Conference of the Astronomische Gesellschaft, Sept. 14–16, 2021, Nuncius Hamburgensis; Beiträge zur Geschichte der Naturwissenschaften, 57, tredition Verlag, Ahrensburg, 775 pp., ISBN 9783347712942, 2022.
Yaskell, S. H.: From research institution to astronomical museum: A history of the Stockholm observatory, Journal of Astronomical History and Heritage, 11, 146–155, 2008.
Yiou, P., Boichu, M., Vautard, R., Vrac, M., Jourdain, S., Garnier, E., Fluteau, F., and Menut, L.: Ensemble meteorological reconstruction using circulation analogues of 1781–1785, Clim. Past, 10, 797–809, https://doi.org/10.5194/cp-10-797-2014, 2014.
Zach, F. X.: Monatliche Korrespondenz, Becker, Gotha, 18, 1808.
Zuidervaart, H. J.: An eighteenth-century medical–meteorological society in the Netherlands an investigation of early organization, instrumentation and quantification. Part 2, Brit. J. Hist. Sci., 39, 49–66, https://doi.org/10.1017/S0007087405007594, 2006.
While Kleinert (2009) could not identify the source with the original quote, Haustein (2004) states Galileo as the source: Il Saggiatore (Galilei, 1623).
The Palatinate was one of seven more important regions of the many princedoms and counties forming Germany during the period of the Holy Roman Empire. Its sovereign was one of the seven “electors”, being empowered to elect the German king.
The speech was held in German but printed in Latin in the first volume of the ephemerides (Hemmer, 1783, 18–23).
On 27 May 1781 Lichtenberg mentioned in a letter (no. 817) to the printer Schwan in Mannheim that he had not obtained the promised instruments. In a third letter to the Scientific Society of Göttingen, dated 29 November 1781 (letter no. 876), he explained not to be able to do the observations due to lack of time and that Gatterer most easily could take over the task.
Gatterer's disregard of Wilse is mentioned in a circulation letter (Lichtenberg, 1985, Vol. II, letter no. 838 of 7 July 1781). Wilse had previously published two books referring to meteorology (Wilse, 1778, 1780), which were known to Gatterer.
Private contacts were also typical in this time and were used for a safe delivery of the tables, e.g. Bernoulli took over meteorological tables from Marseille (letter Bernoulli to secretary Lamey, 15 August 1793 in Generallandesarchiv Karlsruhe, Abt S, Kremer-Lamey, Vol. 133, fol. 189).
Cotte's letter to Lavoisier, cited by Cassidy (1985, footnote 55). Cassidy mentions in footnote 12 that only one of three cases with instruments reached Cotte, but the instruments were broken during control by customs. One of the lost shipments contained the barometer and thermometer, the other the declinometer. However, they reappeared in 1789 in a bureau in Champagne (p. 194).